2.2.2.5 Sub-surface ocean temperatures and salinities
While the upper ocean temperature and salinity are coupled to the atmosphere
on diurnal and seasonal time-scales, the deep ocean responds on much longer
time-scales. During the last decade, data set development, rescue, declassification
and new global surveys have made temperature and salinity profile data more
readily available (Levitus et al., 1994, 2000a).
Global
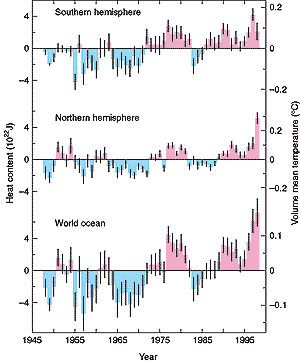
Figure 2.11: Time-series for 1948 to 1998 of ocean heat content
anomalies in the upper 300 m for the two hemispheres and the global
ocean. Note that 1.5 x 1022 J equals 1 watt-year-m-2 averaged
over the entire surface of the earth. Vertical lines through each
yearly estimate are ± one standard error (Levitus et al., 2000b).
|
Levitus et al. (1997, 2000b) made annual estimates of the heat content of the
upper 300 m of the world ocean from 1948 through to 1998 (Figure
2.11). The Atlantic and Indian Oceans each show a similar change from relatively
cold to relatively warm conditions around 1976. The Pacific Ocean exhibits more
of a bidecadal signal in heat storage. In 1998, the upper 300 m of the world
ocean contained (1.0 ± 0.5) x1023 Joules more heat than it
did in the mid-1950s, which represents a warming of 0.3 ± 0.15°C.
A least squares linear regression to the annual temperature anomalies from 1958
to 1998 gives a warming of 0.037°C/decade. White et al. (1997, 1998b) computed
changes in diabatic heat storage within the seasonal mixed layer from 1955 to
1996 between 20°S and 60°N and observed a warming of 0.15 ± 0.02°C
or 0.036°C/decade.
Extension of the analysis to the upper 3,000 m shows that similar changes in
heat content have occurred over intermediate and deep waters in all the basins,
especially in the North and South Atlantic and the South Indian Oceans. The
change in global ocean heat content from the 1950s to the 1990s is equivalent
to a net downwards surface heat flux of 0.3 Wm-2 over the whole period.
Pacific
The winter and spring mixed-layer depths over the sub-tropical gyre of the North
Pacific deepened 30 to 80% over the period 1960 to 1988 (Polovina et al., 1995).
Over the sub-polar gyre, mixed-layer depths reduced by 20 to 30% over the same
period. The surface layer of the sub-polar gyre in the north-east Pacific has
both warmed and freshened, resulting in a lower surface density (Freeland et
al., 1997). Wong et al. (1999) compared trans-Pacific data from the early 1990s
to historical data collected about twenty years earlier. The changes in temperature
and salinity are consistent with surface warming and freshening at mid- and
higher latitudes and the subsequent subduction (downward advection) of these
changes into the thermocline. From 1968/69 and 1990/91, the South Pacific waters
beneath the base of the thermocline have cooled and freshened (Johnson and Orsi,
1997); the greatest cooling and freshening of -1.0°C and 0.25, respectively,
occurred near 48°S and were still observed at 20°S. All the deep water
masses show a cooling and freshening at these high southern latitudes.
Arctic
Recent surveys of the Arctic Ocean (Quadfasel et al., 1993; Carmack et al.,
1995; Jones et al., 1996) have revealed a sub-surface Atlantic-derived warm
water layer that is up to 1°C warmer and whose temperature maximum is up
to 100 dbar shallower than observed from ice camps from the 1950s to the 1980s,
as well as from ice-breaker data in the late 1980s and early 1990s. Warming
is greatest in the Eurasian Basin. Annual surveys of the southern Canada Basin
since 1979 (Melling, 1998), have shown a warming and deepening lower Atlantic
layer, the lower halocline layer cooling by 0.12°C and the upper halocline
layer warming by 0.15°C. Steele and Boyd (1998) compared winter temperature
and salinity profiles obtained over the central and eastern Arctic Basins from
submarine transects in 1995 and 1993 with Soviet data collected over the period
1950 to 1989 (Environmental Working Group, 1997). They showed that the cold
halocline waters cover significantly less area in the newer data. This is consistent
with a decreased supply of cold, fresh halocline waters from the Pacific Shelf
areas.
Atlantic
The sub-arctic North Atlantic exhibits decadal variability in both temperature
and salinity (Belkin et al., 1998). Reverdin et al. (1997) found that the variability
of salinity around the entire subarctic gyre for the period 1948 to 1990 was
most prominent at periods of 10 years and longer, and extended from the surface
to below the base of the winter mixed layer. This salinity signal was only coherent
with elsewhere in the north-western Atlantic. A single spatial pattern explains
70% of the variance of the upper ocean salt content of the subarctic gyre, corresponding
to a signal propagating from the west to the north-east. Reverdin et al. also
found that fluctuations in the outflow of fresh water from the Arctic are associated
with periods of greater or fewer than usual northerly winds east of Greenland
or off the Canadian Archipelago.
North Atlantic deep waters begin as intermediate waters in the Nordic seas.
These waters have freshened over the 1980s and 1990s (Bönisch et al., 1997).
In addition, the absence of deep convection over the same period has caused
Nordic Sea bottom waters to become warmer, saltier and less dense. The Faroes-Shetland
Channel is the principal pathway between the north-east Atlantic and the Norwegian
Sea and has been surveyed regularly since 1893 (Turrell et al., 1999). Unfortunately,
the quality of the salinity measurements was poor from 1930 through to 1960.
Since the mid-1970s, the intermediate and bottom waters entering the North Atlantic
through the channel have freshened at rates of 0.02/decade and 0.01/decade,
respectively. The decreased salinities have resulted in decreased water densities
and a decrease of between 1 and 7%/decade in the transport of deep water into
the North Atlantic.
In the Labrador Sea, winter oceanic deep convection was intense during the earlier
1990s, extending to deeper than 2,400 m in 1992 to 1994. This produced a Labrador
Sea water mass colder, denser and fresher than has been observed over at least
the last five decades (Lazier, 1995; Dickson et al., 1996).
Within the tropical and sub-tropical gyres of the North Atlantic, the deep and
intermediate water masses are warming. Ocean station S (south-east of Bermuda,
32°17'N, 64°50'W) has been sampled bi-weekly since 1954. Joyce and Robins
(1996) extended the hydrographic record from ocean station S back from 1954
to 1922 using nearby observations. They find an almost constant rate of warming
over the 1,500 to 2,500 dbar layer of 0.05°C/decade over the 73-year period
1922 to 1995. This corresponds to a net downward heat flux of 0.7 Wm-2. Sections
completed in 1958, 1985 and 1997 along 52°W and 66°W between 20°N
to 35°N (Joyce et al., 1999) show a rate of warming of 0.06°C/decade,
similar to that seen at Bermuda but averaged over a larger 1,700 m depth interval.
Trans-Atlantic sections along 24°N in 1957, 1981 and 1992 show a similar
warming between 800 and 2,500 m (Parrilla et al., 1994; Bryden et al., 1996).
The maximum warming at 1,100 m is occurring at a rate of 0.1°C/decade. At
8°N between 1957 and 1993, Arhan et al. (1998) showed warming from 1,150
and 2,800 m with the maximum warming of 0.15°C at 1,660 m.
The Antarctic bottom water in the Argentine Basin of the South Atlantic experienced
a marked cooling (0.05°C) and freshening (0.008) during the 1980s (Coles
et al., 1996). The bottom waters of the Vema Channel at the northern end of
the Argentine basin did not change significantly during the 1980s but warmed
steadily during a 700-day set of current meter deployments from 1992 to 1994
(Zenk and Hogg, 1996).
The Indian Ocean
Bindoff and Mcdougall (2000) have examined changes between historical data collected
mostly in the period 1959 to 1966 with WOCE data collected in 1987 in the southern
Indian Ocean at latitudes 30 to 35°S. They found warming throughout the
upper 900 m of the water column (maximum average warming over this section of
0.5°C at 220 dbar).
|