2.2.3 Temperature of the Upper Air
Uncertainties in discerning changes
Several measuring systems are available to estimate the temperature variations
and trends of the air above the surface, though all contain significant time-varying
biases as outlined below.
Weather balloons
The longest data sets of upper air temperature are derived from instruments
carried aloft by balloons (radiosondes). Changes in balloon instrumentation
and data processing over the years have been pervasive, however, resulting in
discontinuities in these temperature records (Gaffen, 1994; Parker and Cox,
1995; Parker et al., 1997). Gaffen et al. (2000b) attempted to identify these
biases by using statistical tests to determine “change-points” –
sudden temperature shifts not likely to be of natural origin (e.g., instrument
changes). However, they found that alternative methods for identifying change
points yield different trend estimates and that the analysis was hampered by
the lack of complete documentation of instrument and data processing changes
for many stations. This study, however, only analysed change points in the time-series
of individual stations in isolation. Another technique, used successfully with
surface data, relies on differences produced from comparisons among several
stations in close proximity. In addition, Santer et al. (1999) noted that temperature
trends estimated from radiosonde data sets are sensitive to how temperature
shifts are dealt with, which stations are utilised, and the method used for
areal averaging.
Worldwide temperatures from the Microwave Sounding Unit (MSU) data (Christy
et al., 2000) have been available from the beginning of 1979 for intercomparison
studies. Parker et al. (1997) used the lower-stratospheric and lower-tropospheric
MSU products to adjust monthly radiosonde reports for stations in Australia
and New Zealand at times when instrumental or data-processing changes were documented.
Some individual stratospheric corrections were as much as 3°C due to radiosonde
instrument changes. The main disadvantage of the Parker et al. technique is
that the raw MSU record has time-varying biases which must first be estimated
and eliminated (Christy et al., 2000).
Gaffen et al. (2000b) compared trends for 1959 to 1995, calculated using linear
regression, for twenty-two stations with nearly complete data records at levels
between 850 and 30 hPa. Each of these stations is included in two data sets
created since the SAR: (a) monthly mean temperatures reported by the weather
balloon station operators (Parker et al., 1997; CLIMAT TEMP data) and (b) monthly
mean temperatures calculated from archived daily weather balloon releases (Eskridge
et al., 1995; CARDS data). Decadal trends at individual sites differed randomly
between the two data sets by typically 0.1°C/decade, with the largest differences
at highest altitudes. In a few cases the differences were larger and statistically
significant at the 1% level. The discrepancies were sometimes traceable to time-of-observation
differences of the data used to calculate the averages.
The analysis of trends requires long station data records with minimal missing
data. The records for 180 stations in the combined Global Climate Observing
System Upper Air Network (GUAN) and the Angell (1988, 2000) network do not generally
meet this standard, as only 74 of the GUAN stations, for instance, have at least
85% of tropospheric monthly means available for 1958 to 1998. In the lower stratosphere
(up to 30 hPa), only twenty-two stations meet this requirement (Gaffen et al.,
2000b). These deficiencies present the dilemma of using either relatively small
networks of stations with adequate data (the Southern Hemisphere, in particular,
is poorly sampled) or larger networks with poorer quality data (adding uncertainty
to the resulting trend estimates).
Characteristics, such as spatial coverage, of each data
set derived from the weather balloon data are different. For example, Sterin
(1999) used data from over 800 stations from the CARDS and telecommunicated
data sets, with only gross spatial and temporal consistency checks. The data
were objectively interpolated to all unobserved regions, introducing extra uncertainty.
Parker et al. (1997) placed CLIMAT TEMP data into 5° latitude x 10°
longitude grid boxes from about 400 sites, leaving unobserved boxes missing.
Further data sets were created employing limited spatial interpolation and bias-adjustments,
but uncertainties related to spatial under-sampling remain (Hurrell et al.,
2000). Angell (1988) placed observations from 63 stations into seven broad latitudinal
bands, calculated the simple average for each band and produced global, hemispheric
and zonal mean anomalies.
Satellites
Radiosondes measure temperatures at discrete levels, but satellite instruments
observe the intensity of radiation from deep atmospheric layers. The advantage
of satellites is the essentially uniform, global, coverage. The three temperature
products that are commonly available from MSU are: the low to mid-troposphere
(MSU 2LT, surface to about 8 km), mid-troposphere (MSU 2, surface to about 18
km, hence including some stratospheric emissions) and the lower stratosphere
(MSU 4, 15 to 23 km, hence including some tropical tropospheric emissions) (Christy
et al., 2000). No other data, such as from radiosondes, are used to construct
these MSU data sets. It is important to note that the troposphere and stratosphere
are two distinct layers of the atmosphere with substantially different temperature
variations and trends. The altitude of the troposphere/stratosphere boundary
varies with latitude, being about 16 to 17 km in the tropics but only 8 to 10
km at high latitudes.
Since the SAR, several issues have emerged regarding MSU temperatures. Mo (1995)
reported that for one of the longest-lived satellites (NOAA-12, 1991 to 1998)
the non-linear calibration coefficients were erroneous, affecting MSU 2 and
MSU 2LT. Wentz and Schabel (1998) discovered that satellite orbit decay introduces
gradual, spurious cooling in MSU 2LT. Christy et al. (1998, 2000) found that
instrument responses often differ between the laboratory assessments and on-orbit
performance, requiring further corrections. Additional adjustments were also
made by recalculating and removing spurious temperature trends due to diurnal
effects induced by the east-west drift of the spacecraft (Christy et al., 2000).
The magnitude of the spurious trends (1979 to 1998) removed from version D compared
to version C were: orbit decay, -0.11; instrument response, +0.04 and diurnal
drift, +0.03°C/decade.
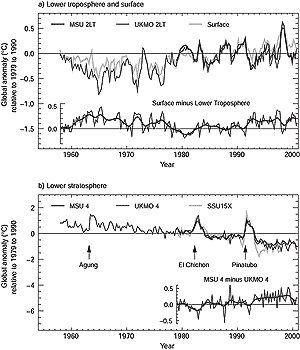
Figure 2.12: (a) Seasonal anomalies of global average temperature
(°C), 1958 to 2000, relative to 1979 to 1990 for the lower troposphere,
as observed from satellites (MSU 2LT) and balloons (UKMO 2LT), and for the
surface (adapted from Jones et al., 2001). Also shown (bottom graph) are
the differences between the surface temperature anomalies and the averages
of the satellite and balloon-based observations of the lower-tropo-spheric
temperature anomalies. (b) As (a) but for the temperature of the lower stratosphere,
as observed from satellites (MSU 4 and SSU 15X) and balloons (UKMO 4). The
times of the major explosive eruptions of the Agung, El Chichon and Mt.
Pinatubo volcanoes are marked. Also shown (bottom graph) are the differences
between the MSU 4 and UKMO 4 based temperature anomalies. |
Version D of the MSU data is used in Figure 2.12.
The SAR presented version B that for the low to mid-troposphere indicated a
global trend about 0.05°C/decade more negative than version D (for 1979
to 1995). Quite separately, Prabhakara et al. (1998) generated a version of
MSU 2 without corrections for satellite drift or instrument body effects, in
many ways similar to MSU 2 version A of Spencer and Christy (1992a, 1992b).
The Stratospheric Sounding Unit (SSU) detects the intensity of thermal emissions
and measures deep layer temperatures at altitudes above 20 km (Nash and Forrester,
1986). As with the MSU products, adjustments are required for radiometer biases,
diurnal sampling and orbital drift (Chanin and Ramaswamy, 1999).
Rocketsondes and lidar
Data sets generated from rocketsondes have been updated (Golitsyn et al., 1996;
Lysenko et al., 1997), providing temperature information to as high as 75 km.
Important difficulties arise with these data due to different types of instrumentation,
tidal cycles (amplitude 2°C) and to assumed corrections for aerodynamic
heating. The last set of adjustments has the most significant impact on trends.
The approximately 11-year solar cycle forces a temperature perturbation of >1°C
in the mid- to upper stratosphere (30 to 50 km). Keckhut et al. (1999) and Dunkerton
et al. (1998) created a quality-controlled data set of these measurements, which
is used in Chanin and Ramaswamy (1999). The very limited number of launch sites
leads to some uncertainty in deduced temperatures, and most launches were terminated
in the mid-1990s.
Rayleigh lidar measurements began in 1979 at the Haute Provence Observatory
in southern France and have spread to locations around the world. Lidar techniques
generate the vertical profile of temperature from 30 to 90 km, providing absolute
temperatures within 2.5°C accuracy. Chanin and Ramaswamy (1999) have combined
MSU 4, SSU, radiosonde, lidar and rocketsonde data to estimate 5-km thick layer
temperature variations for altitudes of 15 to 50 km, generally limited to the
Northern Hemisphere mid-latitudes.
Reanalyses
The principle of reanalysis is to use observations in the data assimilation
scheme of a fixed global weather forecasting model to create a dynamically consistent
set of historical atmospheric analyses (Kalnay et al., 1996). Within the assimilation
scheme, potentially errant data are amended or excluded using comparisons with
neighbours and/or calculated conditions. However, small, time-dependent biases
in the observations, of magnitudes important for climate change, are virtually
impossible to detect in the model, even in areas of adequate in situ data. Furthermore,
in areas with few in situ data the reanalyses are often affected by inadequate
model physics or satellite data for which time-varying biases have not been
removed.
Though interannual variability is reproduced well, known discontinuities in
reanalysed data sets indicate that further research is required to reduce time-dependent
errors to a level suitable for climate change studies (Basist and Chelliah,
1997; Hurrell and Trenberth, 1998; Santer et al., 1999, 2000; Stendel et al.,
2000). It is anticipated that future assessments of climate change will utilise
reanalysis products to which substantial improvements will have been made. Data
from the NCEP reanalysis are included below for comparison purposes, but longer-term
stratospheric trends from NCEP are especially suspect due to a large shift in
temperature when satellite data were incorporated for the first time in 1978
(Santer et al., 1999).
|