8.5.5 Past Climates
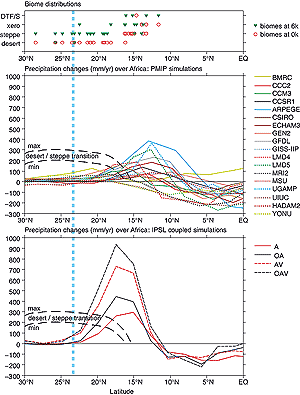
Figure 8.13: Annual mean precipitation changes (mm/yr) over Africa
(20°W to 30°E) for the mid-Holocene climate: (upper panel) Biome
distributions (desert, steppe, xerophytic and dry tropical forest/savannah
(DTF/S)) as a function of latitude for present (red circles) and 6,000 yr
BP (green triangles), showing that steppe vegetation replaces desert at
6,000 yr BP as far north as 23°N (vertical blue dashed line); (middle
panel) 6000 yr BP minus present changes as simulated by the PMIP models.
The black hatched lines are estimated upper and lower bounds for the excess
precipitation required to support grassland, based on present climatic limits
of desert and grassland taxa in palaeo-ecological records, the intersection
with the blue vertical line indicates that an increase of 200 to 300 mm/yr
is required to sustain steppe vegetation at 23°N at 6,000 yr BP (redrawn
from Joussaume et al., 1999); (lower panel) same changes for the IPSL atmosphere-alone
(A), i.e., PMIP simulation, the coupled atmosphere-ocean (OA), the atmosphere-alone
with vegetation changes from OA (AV) and the coupled atmosphere-ocean-vegetation
(OAV) simulations performed with the IPSL coupled climate model. The comparison
between AV and OAV emphasises the synergism between ocean and land feedbacks
(redrawn from Braconnot et al., 1999). |
Accurate simulation of current climate does not
guarantee the ability of a model to simulate climate change correctly. Climate
models now have some skill in simulating changes in climate since 1850 (see
Section 8.6.1), but these changes are fairly small compared with many projections
of climate change into the 21st century. An important motivation for attempting
to simulate the climatic conditions of the past is that such experiments provide
opportunities for evaluating how models respond to large changes in forcing.
Following the pioneering work of the Co-operative Holocene Mapping Project (COHMAP-Members,
1988), the Paleoclimate Modeling Intercomparison Project (PMIP) (Joussaume and
Taylor, 1995; PMIP, 2000) has fostered the more systematic evaluation of climate
models under conditions during the relatively well-documented past 20,000 years.
The mid-Holocene (6,000 years BP) was chosen to test the response of climate
models to orbital forcing with CO2 at pre-industrial concentration and present
ice sheets. The orbital configuration intensifies (weakens) the seasonal distribution
of the incoming solar radiation in the Northern (Southern) Hemisphere, by about
5% (e.g., 20 Wm-2 in the boreal summer). The last glacial maximum (21,000 years
BP) was chosen to test the response to extreme cold conditions
8.5.5.1 Mid-Holocene
Atmosphere alone simulations
Within PMIP, eighteen different atmospheric general circulation models using
different resolutions and parametrizations have been run under the same mid-Holocene
conditions, assuming present-day conditions over the oceans (Joussaume et al.,
1999). In summer, all of the models simulate an increase and northward expansion
of the African monsoon; conditions warmer than present in high northern latitudes,
and drier than present in the interior of the northern continents. Palaeo-data
do not support drying in interior Eurasia (Harrison et al., 1996; Yu and Harrison,
1996; Tarasov et al., 1998), but they clearly show an expanded monsoon in northern
Africa (Street-Perrott and Perrott 1993; Hoelzmann et al., 1998; Jolly et al.,
1998a, 1998b), warming in the Arctic (Texier et al., 1997), and drying in interior
North America (Webb et al., 1993).
Vegetation changes reconstructed from pollen data in the BIOME 6000 project
(Jolly et al., 1998b; Prentice and Webb III, 1998) provide a quantitative model-data
comparison in northern Africa. The PMIP simulations produce a northward displacement
of the desert-steppe transition, qualitatively consistent with biomes, but strongly
underestimated in extent (Harrison et al., 1998). At least an additional 100
mm/yr of precipitation would be required for most models to sustain grassland
at 23°N, i.e., more than twice as much as simulated in this area (Joussaume
et al., 1999) (Figure 8.13). The increased area of lakes
in the Sahara has also been quantified (Hoelzmann et al., 1998) and, although
the PMIP simulations do produce an increase, this latter is not large enough
(Coe and Harrison, 2000). A similar underestimation is obtained at high latitudes
over northern Eurasia, where PMIP simulations produce a northward shift of the
Arctic tree-line in agreement with observed shifts (Tarasov et al., 1998) but
strongly underestimated in extent (Kutzbach et al., 1996b; Texier et al., 1997;
Harrison et al., 1998). Model-data discrepancies may, however, be due to missing
feedbacks in the simplified PMIP experimental design.
Ocean feedbacks
Recent experiments with asynchronous (Kutzbach and Liu 1997; Liu et al., 1999)
and synchronous (Hewitt and Mitchell, 1998; Braconnot et al., 2000) coupling
of atmospheric models to full dynamical ocean models have been performed for
the mid-Holocene. They all produce a larger enhancement of the African monsoon
than shown in their PMIP atmosphere only experiments, resulting from the ocean
thermal inertia and changes in the meridional ocean heat transport (Braconnot
et al., 2000). However, the changes are not sufficient to reproduce the observed
changes in biome shifts over northern Africa.
Coupled models are also beginning to address the issue of changes in interannual
to inter-decadal variability under conditions of large differences in the basic
climate. Some palaeo-environmental evidence has suggested that short-term climate
variability associated with El Niño-Southern Oscillation (ENSO) was reduced
during the early to mid-Holocene (Sandweiss et al., 1996; Rodbell, 1999). Up
to now, ENSO variability has only been analysed in the CSM simulation, exhibiting
no significant change at the mid-Holocene (Otto-Bliesner, 1999).
Land-surface feedbacks
Land-surface changes also provide an additional important feedback. During the
mid-Holocene, vegetation changes over northern Africa have indeed favoured a
larger increase in monsoon precipitation as shown through sensitivity experiments
(Kutzbach et al., 1996a; Brostrom et al., 1998; Texier et al., 2000) as well
as through coupled atmosphere-vegetation experiments (Claussen and Gayler, 1997;
Texier et al., 1997; Pollard et al., 1998; Doherty et al., 2000; de Noblet et
al., 2000). Including the observed occurrence of large lakes and wetlands (Coe
and Bonan, 1997; Brostrom et al., 1998) also intensifies monsoon rains. Vegetation
feedbacks also amplify the effects of orbital forcing at high latitudes where
they led to greater and more realistic shifts of vegetation cover over northern
Eurasia (Foley et al., 1994; Kutzbach et al., 1996b; Texier et al., 1997). The
importance of land-surface feedbacks has further been emphasised in the IPSL
AOGCM coupled to a vegetation model (Braconnot et al., 1999). The IPSL simulation
shows that combined feedbacks between land and ocean lead to a closer agreement
with palaeo-data (Figure 8.13). The ocean feedback increases
the supply of water vapour, while the vegetation feedback increases local moisture
recycling and the length of the monsoon season. The importance of land-surface
feedbacks has also been shown by an EMIC (Ganopolski et al., 1998a), further
emphasising that vegetation feedbacks may explain abrupt changes in Saharan
vegetation in the mid-Holocene (Claussen et al., 1999).
|