11.2.2 Glaciers and Ice Caps
Box 11.2: Mass balance terms for glaciers, ice caps
and ice sheets
A glacier, ice cap or ice sheet gains mass by accumulation of snow (snowfall
and deposition by wind-drift), which is gradually transformed to ice,
and loses mass (ablation) mainly by melting at the surface or base with
subsequent runoff or evaporation of the melt water. Some melt water may
refreeze within the snow instead of being lost, and some snow may sublimate
or be blown off the surface. Ice may also be removed by discharge into
a floating ice shelf or glacier tongue, from which it is lost by basal
melting and calving of icebergs. Net accumulation occurs at higher altitude,
net ablation at lower altitude; to compensate for net accumulation and
ablation, ice flows downhill by internal deformation of the ice and sliding
and bed deformation at the base. The rate at which this occurs is mainly
controlled by the surface slope, the ice thickness, the effective ice
viscosity, and basal thermal and physical conditions. The mass balance
for an individual body of ice is usually expressed as the rate of change
of the equivalent volume of liquid water, in m3/yr; the mass balance is
zero for a steady state. Mass balances are computed for both the whole
year and individual seasons; the winter mass balance mostly measures accumulation,
the summer, surface melting. The specific mass balance is the mass balance
averaged over the surface area, in m/yr. A mass balance sensitivity is
the derivative of the specific mass balance with respect to a climate
parameter which affects it. For instance, a mass balance sensitivity to
temperature is in m/yr/°C.
|
11.2.2.1 Mass balance studies
The water contained in glaciers and ice caps (excluding the ice sheets of Antarctica
and Greenland) is equivalent to about 0.5 m of global sea level (Table 11.3).
Glaciers and ice caps are rather sensitive to climate change; rapid changes
in their mass are possible, and are capable of producing an important contribution
to the rate of sea level rise. To evaluate this contribution, we need to know
the rate of change of total glacier mass. Unfortunately sufficient measurements
exist to determine the mass balance (see Box 11.2 for definition) for only a
small minority of the world’s 105 glaciers.
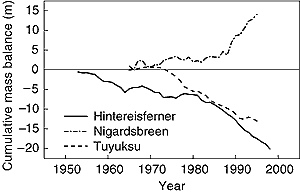
Figure 11.2: Cumulative mass balance for 1952-1998 for three glaciers
in different climatic regimes: Hintereisferner (Austrian Alps), Nigardsbreen
(Norway), Tuyuksu (Tien Shan, Kazakhstan). |
A possible approximate approach to this problem is to
group glaciers into climatic regions, assuming glaciers in the same region to
have a similar specific mass balance. With this method, we need to know only
the specific mass balance for a typical glacier in each region (Kuhn et al.,
1999) and the total glacier area of the region. Multiplying these together gives
the rate of change of glacier mass in the region. We then sum over all regions.
In the past decade, estimates of the regional totals of area and volume have
been improved by the application of high resolution remote sensing and, to a
lesser extent, by radio-echo-sounding. New glacier inventories have been published
for central Asia and the former Soviet Union (Dolgushin and Osipova, 1989; Liu
et al., 1992; Kuzmichenok, 1993; Shi et al., 1994; Liu and Xie, 2000; Qin et
al., 2000), New Zealand (Chinn, 1991), India (Kaul, 1999) South America (Casassa,
1995; Hastenrath and Ames, 1995; Skvarca et al., Aniya et al, 1997; Kaser, 1999;
1995; Kaser et al., 1996; Rott et al., 1998), and new estimates made for glaciers
in Antarctica and Greenland apart from the ice sheets (Weidick and Morris, 1996).
By contrast, specific mass balance is poorly known. Continuous mass balance
records longer than 20 years exist for about forty glaciers worldwide, and about
100 have records of more than five years (Dyurgerov and Meier, 1997a). Very
few have both winter and summer balances; these data are critical to relating
glacier change to climatic elements (Dyurgerov and Meier, 1999). Although mass
balance is being monitored on several dozen glaciers worldwide, these are mostly
small (<20 km2) and not representative of the size class that
contains the majority of the mass (>100 km2). The geographical
coverage is also seriously deficient; in particular, we are lacking information
on the most important maritime glacier areas. Specific mass balance exhibits
wide variation geographically and over time (Figure 11.2). While glaciers in
most parts of the world have had negative mass balance in the past 20 years,
glaciers in New Zealand (Chinn, 1999; Lamont et al., 1999) and southern Scandinavia
(Tvede and Laumann, 1997) have been advancing, presumably following changes
in the regional climate.
Table 11.4: Estimates of historical contribution
of glaciers to global average sea level rise. |
 |
Reference |
Period |
Rate of sea-level rise (mm/yr) |
Remarks |
Meier (1984) |
1900 to 1961 |
|
|
Trupin et al. (1992) |
1965 to 1984 |
|
|
Meier (1993) |
1900 to 1961 |
|
|
Zuo and Oerlemans (1997), Oerlemans (1999) |
1865 to 1990 |
0.22 0.07a |
Observeed temperature changes with mass balance |
1961 to 1990 |
0.3a |
Dyurgeov and Meier (1997b) |
1961 to 1990 |
0.25 0.10 |
Area-weighted mean of observed mass balance for seven regions |
Dowdeswell et al. (1997) |
1945 to 1995 approx |
0.13 |
Observed mass balance, Arctic only |
Gregory and Oerlemans (1998) |
1860 to 1990 |
0.15a |
General Circulation Model (GCM) temperature changes with
mass balance sensitivities from Zuo and Oerlemans (1997) |
1960 to 1990 |
0.26a |
 |
Estimates of the historical global glacier contribution to sea level rise are
shown in Table 11.4. Dyurgerov and Meier (1997a) obtained their estimate by
dividing a large sample of measured glaciers into seven major regions and finding
the mass balance for each region, including the glaciers around the ice sheets.
Their area-weighted average for 1961 to 1990 was equivalent to 0.25 ±
0.10 mm/yr of sea level rise. Cogley and Adams (1998) estimated a lower rate
for 1961 to 1990. However, their results may be not be representative of the
global average because they do not make a correction for the regional biases
in the sample of well investigated glaciers (Oerlemans, 1999). When evaluating
data based on observed mass balance, one should note a worldwide glacier retreat
following the high stand of the middle 19th century and subsequent small regional
readvances around 1920 and 1980.
|