5.7.3.1.2. Direct use of water
Intensification in direct uses of water will negatively influence "natural"
waters and could seriously reduce services provided by these ecosystems. Some
values and services of lakes and rivers would be degraded by human responses
to their greater demands for water for direct human use through dam construction,
dyke and levee construction, water diversions, and wetland drainage (Postel
and Carpenter, 1997). Such activities can alter water temperatures, recreation,
pollution dilution, hydropower, transportation, and the timing and quantity
of river flows; lower water levels; destroy the hydrologic connection between
the river and the river floodplain; reduce natural flood control, nutrient and
sediment transport, and delta replenishment; eliminate key components of aquatic
environments; and block fish migration. At risk are aquatic habitat, biodiversity
habitat, sport and commercial fisheries, waterfowl, natural water filtration,
natural floodplain fertility, natural flood control, and maintenance of deltas
and their economies (Ewel, 1997).
Water-level increases as well as decreases can have negative influences on
lakes and streams. A recent example is from Lake Baikal, where the combined
influences of a dam on the outlet and increased precipitation has increased
water level by 1.5 m and decreased biodiversity and fishery production (Izrael
et al., 1997; ICRF, 1998).
5.7.3.2. Fisheries and Biodiversity
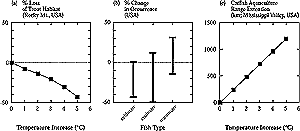
Figure 5-7: Simulated changes in thermal habitat for fish in the continental
United States. Left panel is modified from Keleher and Rahel (1996). Center
panel is modified from Mohseni and Stefan (2000) and Fang et al.(1998).
Right panel is modified from McCauley and Beitinger (1992). Simulation is
for a 2xCO2 climate from the Canadian Climate Model and represents
air temperature increases of 3-6.5ºC in different parts of the
United States. Coldwater fish include trout and salmon; coolwater fish include
yellow perch, walleye, northern pike, and white sucker; and warmwater fish
include sunfish (black basses, bluegill, pumpkinseed) and common carp. |
Potential effects of climate change on fisheries are documented
in the SAR by Everett et al. (1996); freshwater fisheries in small rivers and
lakes are considered to be more sensitive to changes in temperature and precipitation
than those in large lakes and rivers. Changes in temperature and precipitation
resulting from climate change are likely to have direct impacts on freshwater
fisheries through changes in abundance, distribution, and species composition.
Because current exploitation rates tend to be high or excessive, any impact
that concentrates fish will increase their catchability and further stress the
population.
Assessing the potential impacts of climate change on aquaculture is uncertain,
in part because the aquaculture industry is mobile and in a period of rapid
expansion. There is no question that large abundances relative to traditional
wild harvests can be produced in small areas and that in many cases these sites
can be moved to more favorable locations. Changes in groundwater may be especially
significant for aquaculture. In tropical areas, crustaceans are cultured in
ponds (Thia-Eng and Paw, 1989); fish frequently are cultured in cages. Commonly
cultured species such as carp and tilapia may grow faster at elevated temperatures,
but more food is required and there is an increased risk of disease. Temperature
is a key factor affecting growth, but other factors relating to water quality
and food availability can be important. Modeling studies suggest that for every
1°C average increase, the rate of growth of channel catfish would increase
by about 7%, and the most favorable areas for culture would move 240 km northward
in North America (McCauley and Beitinger, 1992; see Figure
5-7, right panel). The southern boundary also would move northward as surface
water temperatures increase, perhaps exceeding lethal ranges on occasion. Growth
would increase from about 13 to 30°C and then fall off rapidly as the upper
lethal limit of about 35°C is reached (McCauley and Beitinger, 1992). Thus,
aquaculture for traditional species at a specific location may have to switch
to warmer water species.
Warmer conditions are more suitable for warmer loving flora and fauna and less
suitable for cold-loving flora and fauna. Warmer temperatures, however, lead
to higher metabolic rates, and if productivity of prey species does not increase,
reductions in growth would occur at warmer temperatures (Arnell et al., 1996;
Magnuson et al., 1997; Rouse et al., 1997). Rates of natural dispersal across
land barriers of less mobile species poleward or to higher altitudes are not
likely to keep up with rates of change in freshwater habitats. Species most
affected would include fish and mollusks; in contrast, almost all aquatic insects
have an aerial life history stage, thus are less likely to be restricted. Some
streams have a limited extent and would facilitate only limited poleward or
altitudinal dispersal. This could be especially problematic as impoundments
restricting movements of organisms increase in number. Coldwater species and
many coolwater species would be expected to be extirpated or go extinct in reaches
where temperatures are at the warmer limits of a species range. Many lakes do
not have surface water connections to adjacent waters, especially in headwater
regions where interlake movement would be limited without human transport of
organisms across watershed boundaries.
Exotics will become a more serious problem for lake and stream ecosystems with
warming. In the northern hemisphere, for example, range extensions occur along
the northern boundaries of species ranges and extinctions occur along the southern
boundaries, in natural waters and in aquaculture operations (Arnell et al.,
1996; Magnuson et al., 1997). In addition, loss of habitat for biodiversity
will result from warmer, drier conditions interacting with increases in impoundment
construction.
Distributions of fish are simulated to move poleward across North America and
northern Europe. In northern Europe, Lehtonen (1996) forecasts a shrinking range
for 11 coldwater species and an expanding range for 16 cool- and warmwater fish.
In simulations for Finland by Lappalainen and Lehtonen (1995), lake whitefish
lost habitats progressively toward the north, whereas brown trout did better,
at least in the north. Coolwater fish were forecast to spread northward through
the country. In simulation studies, boundaries of individual warmwater species
ranges in were projected to move northward by 400-500 km in Ontario, Canada
(Minns and Moore, 1995), and southern boundaries of coldwater fishes were projected
to move 500-600 km northward in the southeastern United States (estimated
from Fang et al., 1998). In simulations based on an elevated CO2
scenario using the Canadian Climate Centre model, warmwater fish were projected
to benefit in shallow eutrophic and mesotrophic lakes around the United States,
owing to reduction in winterkill, but habitable lakes and streams for coolwater
fish and especially coldwater fish were projected to decline, owing to summer
kill (Fang et al., 1998; Mohseni and Stefan, 2000). Habitat changes from various
studies over large regions (see Figure 5-7, center panel)
projected 0-43% reductions for coldwater species, 50% reductions to 12%
increases for coolwater species, and 14% reductions to 31% increases for warmwater
fish. Changes differ among ecosystem types and areas, depending on latitude
and altitude.
A dramatic picture of the regional decline in trout habitat in the Rocky Mountain
region of the western United States is provided by Keleher and Rahel (1996)
(see Figure 5-7, left panel). Even a 1°C increase in
mean July air temperatures is simulated to decrease the length of streams inhabitable
by salmonid fish by 8%; a 2°C increase causes a reduction of 14%, a 3°C
increase causes a 21% decline, a 4°C increase causes a 31% reduction, and
a 5°C increase causes a 43% reduction. There also is likely to be a increased
fragmentation of inhabitable areas for the North Platte River Drainage in Wyoming
(Rahel et al., 1996).
|