12.1.5. Climate Scenarios Used in Regional Studies
12.1.5.1. Spatial Patterns of Temperature and Rainfall
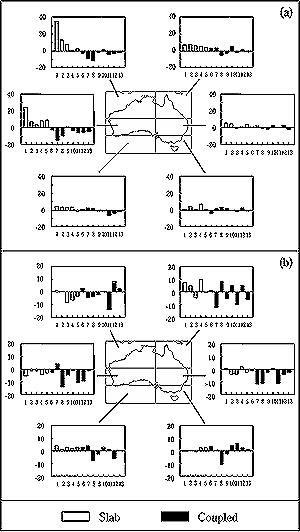
Figure 12-1: Enhanced greenhouse changes in rainfall (as % change
per °C of global warming) for five slab-ocean GCM simulations (open
bars) and eight coupled ocean-atmosphere GCM simulations (full bars), for
six subregions of Australia in (a) summer (DJF) and (b) winter (JJA) Source:
Whetton et al., 2001 (where individual models are identified). Spatial patterns
and scatter plots are given for most of the same coupled models in Hulme
and Sheard (1999) and Carter et al. (2000). |
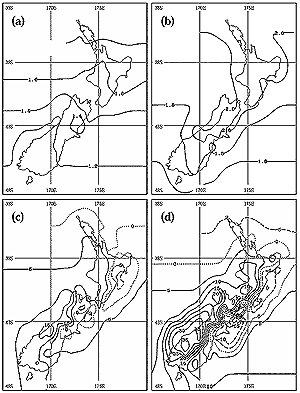
Figure 12-2: Scenarios for changes from the 1980s to the 2080s for
New Zealand: (a) mean summer (DJF) temperature (°C), (b) mean winter
(JJA) temperature (°C), (c) summer precipitation (%), and (d) winter
precipitation (%). These plots were derived by averaging downscaled projections
from four GCMs (CCC, CCSR, CSIRO9, and HadCM2) driven by CO2
concentrations increasing at a compound rate of 1% yr-1, plus
specified sulfate aerosol concentrations (Mullan et al., 2001). |
Most recent impact studies for Australia and New Zealand
have been based on scenarios released by the Commonwealth Scientific and Industrial
Research Organisation (CSIRO, 1996a) or the National Institute of Water and
Atmosphere (NIWARenwick et al., 1998b). The CSIRO (1996a) scenarios
included two sets of rainfall scenarios that are based on results from equilibrium
slab-ocean general circulation model (GCM) and transient coupled ocean-atmosphere
GCM (AOGCM) simulations. Some impact and adaptation studies since RICC have
used results from a 140-year simulation over the whole region that uses the
CSIRO regional climate model (RCM) at 125-km resolution nested in the CSIRO
Mark 2 coupled GCM run in transient mode from 1961 to 2100, as well as similar
simulations at 60-km resolution over eastern Australia. Figure
12-1 (from Whetton et al., 2001) summarizes results of slab-ocean
and coupled AOGCM simulations of rainfall changes for various subregions of
Australia in summer (December-January-February, DJF) and winter (JJA), respectively.
Figures 12-1a and 12-1b clearly
show a variety of estimates for different model simulations; nevertheless, there
is a tendency toward more decreases in winter rainfall in the southwest and
the central eastern coast of Australia in the coupled model results than in
the slab-ocean model results and a similar tendency for more agreement between
coupled model results on less rainfall in the northwest and central west in
summer.
In line with WGI conclusions, more recent scenarios, such as those of Hulme
and Sheard (1999) and Carter et al. (2000) rely exclusively on coupled
models; they also are based on the newer emissions scenarios from the IPCC's
Special Report on Emissions Scenarios (SRES) rather than the IS92 scenarios
described in IPCC (1996). This leads to some changes in the regional scenarios.
According to Whetton (1999), the CSIRO (1996a) scenarios give warmings of 0.3-1.4°C
in 2030 and 0.6-3.8°C in 2070 relative to 1990, compared to estimates
by Hulme and Sheard (1999) of 0.8-3.9°C by the 2050s and 1.0-5.9°C
in the 2080s, relative to the 1961-1990 averages. As discussed by Whetton
(1999), both sets of scenarios use results from several coupled models, but
the use of the SRES emissions scenarios leads to greater warmings in Hulme and
Sheard (1999) than those that are based on the IS92 emission scenarios in CSIRO
(1996). Nevertheless, the Hulme and Sheard (1999) results are preliminary in
that they use scaled results from non-SRES simulations, rather than actual GCM
simulations with SRES emissions.
Rainfall scenarios that are based on the coupled model results in CSIRO (1996a)
show decreases of 0-8% in 2030 and 0-20% in 2070, except in southern
Victoria and Tasmania in winter and eastern Australia in summer, where rainfall
changes by -4 to +4% in 2030 and -10 to +10% in 2070. The Hulme and
Sheard (1999) scenarios suggest that annual precipitation averaged over either
northern or southern Australia is likely to change by -25 to +5%. Larger
decreases are indicated for the 2080s and for some specific locations (e.g.,
a 50% decrease in parts of the southwest of western Australia in winter). This
last finding suggests that even though the rainfall decrease in this region
in the late 20th century was almost certainly dominated by natural variability
(Smith et al., 2000), a decreasing trend resulting from the enhanced
greenhouse effect may dominate in this region by the mid- to late 21st century.
It is important to note that changing from joint use of equilibrium slab-ocean
GCM and transient coupled AOGCM results to exclusive reliance on coupled model
results in the Australian scenarios leads to a marked narrowing of the uncertainty
range in rainfall changes predicted for southern Australia, with a tendency
to more negative changes on the mainland (see Figure 12-1;
Hulme and Sheard, 1999; Carter et al., 2000). This means that the results
of impact studies that used the wider range of rainfall scenarios, as in the
CSIRO (1996a) scenarios (e.g., Schreider et al., 1996), should be reinterpreted
to focus on the drier end of the previous range. This is consistent with more
recent studies by Kothavala (1999) and Arnell (1999).
To summarize the rainfall results, drier conditions are anticipated for most
of Australia over the 21st century. However, consistent with conclusions in
WGI, an increase in heavy rainfall also is projected, even in regions with small
decreases in mean rainfall. This is a result of a shift in the frequency distribution
of daily rainfall toward fewer light and moderate events and more heavy events.
This could lead to more droughts and more floods.
Recent Australian impact studies have tended to use regional scenarios of temperature
and rainfall changes per degree of global warming, based on the CSIRO RCM transient
simulations, scaled to the range of uncertainty of the global warming derived
from the IPCC Second Assessment Report (SAR) range of scenarios. For example,
Hennessy et al. (1998) gives scenarios for six regions of New South Wales
(NSW), based on a 60-km resolution simulation. Ranges are given for estimated
changes in maximum and minimum temperatures, summer days over 35°C, winter
days below 0°C, seasonal mean rainfall changes, and numbers of extremely
wet or dry seasons per decade. Statistical downscaling (discussed in detail
in et al. 3) has not been used extensively in Australia apart from work
by Charles et al. ( 1999) on the southwest of western Australia. It should
be noted that in some cases, RCM results may change the sign of rainfall changes
derived from coarser resolution GCMs, because of local topographic and other
effects (Whetton et al., 2001).
New Zealand scenarios reported in RICC (Basher et al., 1998) are based
on statistical downscaling from equilibrium GCM runs with CO2 held
constant at twice its present concentration. Renwick et al. (1998b, 2000)
also have downscaled equilibrium GCM simulations over New Zealand, using a nested
RCM.
A key factor in rainfall scenarios for New Zealand is the strength of the mid-latitude
westerlies because of the strong orographic influence of the backbone mountain
ranges lying across this flow (Wratt et al., 1996). Earlier equilibrium
slab-ocean GCM runs (applicable only long after stabilization of climate change)
and the regional simulations obtained by downscaling them through statistical
and nested modeling techniques predict a weakening of the westerlies across
New Zealand, particularly in winter. As a result, downscaled equilibrium model
runs predict that winter precipitation will remain constant or decrease slightly
in the west but increase in Otago and Southland (Whetton et al., 1996a;
Renwick et al., 2000). In contrast, transient coupled AOGCM runs suggest
that over the next 100 years or more, the mean strength of the westerlies actually
will increase in the New Zealand region, particularly in winter (Whetton et
al., 1996a; Russell and Rind, 1999; Mpelasoka et al., 2001). As a
result, downscaled transient model results predict that rainfalls will increase
in the west of New Zealand but decrease in the east (Mullan et al., 2001)
as shown in Figure 12-2. Slightly larger temperature increases
are predicted in the north of the country than in the south. Projected temporal
changes in the westerlies before and after stabilization of climate also are
likely to influence rainfall in southern Australia, especially in Tasmania.
GCM simulations extending beyond the stabilization of GHG concentrations indicate
that global warming continues for centuries after stabilization of concentrations
(Wigley, 1995; Whetton et al., 1998; TAR WGI Chapters
9 and 11) but at a much reduced rate as the oceans gradually
catch up with the stabilized radiative forcing. Importantly for the Australasian
region, simulated patterns of warming and rainfall changes in the southern hemisphere
change dramatically after stabilization of GHG concentrations. This is because
of a reversal of the lag in warming of the Southern Ocean relative to the rest
of the globe. This lag increases up to stabilization but decreases after stabilization
(Whetton et al., 1998), with consequent reversal of changes in the north-south
temperature gradient, the mid-latitude westerlies, and associated rainfall patterns.
|