12.6. Settlements and Industry
12.6.1 Infrastructure
Climate change will affect settlements and industry through changes in mean
climate and changes in the frequency and intensity of extreme events. Obviously,
changes in average climate affect design and performance, including variables
such as heating and cooling demand, drainage, structural standards, and so forth.
However, in many cases average climate is only a proxy for design standards
that are developed to cope with extreme demands or stresses such as flooding
rains, gale-force wind gusts, heat waves, and cold spells.
If the severity, frequency, or geographic spread of extreme events changes,
the impact of such changes on infrastructure may be severe. For instance, movement
of tropical cyclones further south into areas where infrastructure is not designed
to cope with them would have significant consequences.
The rate and nature of degradation of infrastructure is directly related to
climatic factors. Computer models to predict degradation as a function of location,
materials, and design and construction factors have been developed (Cole et
al., 1999a,b,c,d). Because buildings and infrastructure that are being constructed
now will have projected lives until 2050, placement, design, and construction
changes to guarantee this life against climate change are needed. Moreover,
the effects of degradation and severe meteorological events may have an unfortunate
synergy. Increase in the rate of degradation as a result of climate change may
promote additional failures when a severe event occurs. If the intensity or
geographical spread of severe events changes, this effect may be compounded.
In New Zealand, a Climate Change Sustainability Index (CCSI) developed by Robinson
(1999) rates the impact of climate change on a house and the contribution to
climate change of GHG emissions from the house. The index includes GHG emissions
from heating and cooling, comfort, tropical cyclone risk, and coastal and inland
flooding. Basically, the closer the house is to sea level and/or a river or
waterway, the lower the CCSI. Higher temperatures and rainfalls in general will
shorten the life span of many buildings.
The impact of extreme climatic events already is very costly in both countries.
This has been documented for Australia in Pittock et al. (1999), where it is
shown that major causes of damage are hail, floods, tropical cyclones, and wildfire.
In New Zealand, floods and landslides are the most costly climatically induced
events, with strong winds and hail also important. The International Federation
of Red Cross and Red Crescent Societies (1999) report estimates damages from
a combination of drought, flood, and high wind (including cyclones, storms,
and tornadoes) in Oceania (Australia, New Zealand, and the Pacific islands)
to be about US$870 million yr-1 over the years 1988-1997. This figure apparently
does not include hail damage, which is a major cost. Insured losses from a single
severe hailstorm that struck Sydney in April 1999 were estimated at about AU$1.5
billion (roughly US$1 billion) (NHRC, 1999).
A scoping study for Queensland Transport (Queensland Transport et al., 1999)
has identified vulnerabilities for the Queensland transport infrastructure that
will require adaptation. Infrastructure considered include coastal highways
and railways, port installations and operations (as a result of high winds,
sea-level rise, and storm surges), inland railways and roads (washouts and high
temperatures), and some airports in low-lying areas. Key climate variables considered
were extreme rainfall, winds, temperatures, storm surge, flood frequency and
severity, sea waves, and sea level. Three weather systems combining extremes
of several of these variablestropical cyclones, east coast lows, and tropical
depressionsalso were assessed.
Regional projections for each of these variables were created, with levels
of confidence, for four regions of Queensland for 2030, 2070, and 2100. Overall,
the potential effects of climate change were assessed as noticeable by 2030
and likely to pose significant risks to transport infrastructure by 2070, if
no adaptation were undertaken. Setting new standards, in the form of new design
criteria and carrying out specific assessments in prioritized areas where infrastructure
is vulnerable, was recommended for roads and rail under threat of flooding,
bridges, and ports. Detailed risk assessments for airports in low-lying coastal
locations were recommended.
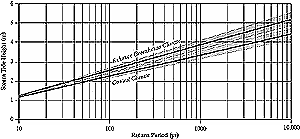
Figure 12-4: Simulated return periods (average time between events)
of storm tides in Cairns, Queensland, for present climate (lower curve),
and for enhanced greenhouse climate (upper curve), assuming 10 hPa lowering
of central pressures and increased variability (additional 5 hPa standard
deviation) of tropical cyclones. Anticipated mean sea-level rise should
be added to these estimates. Uncertainty ranges of simulations are shown
via grey shading (Walsh et al., 2000). |
Many ports and coastal communities already suffer from occasional storm-surge
flooding and wave damage. A series of studies identifies particular vulnerabilities
in some Queensland coastal cities (Smith and Greenaway, 1994; AGSO, 1999). Inland
and coastal communities also are vulnerable to riverine flooding (Smith et al.,
1997; Smith, 1998a). A key feature of these studies is the nonlinear nature
of damage response curves to increased magnitude and frequency of extreme events.
This is partly because of exceedance of present design standards and the generally
nonlinear nature of the damage/stress relationship, with the onset of building
collapse and chain events from flying or floating debris (Smith, 1998a).
A study by McInnes et al. (2000; see also Walsh et al., 2000) estimates the
height of storm tides at the city of Cairns, in northern Queensland, for the
present climate and for an enhanced greenhouse climate in whichbased on
the findings of Walsh and Ryan (2000)the central pressure of tropical
cyclones was lowered by about 10 hPa and the standard deviation of central pressure
(a measure of variability) was increased by 5 hPa but the numbers were unchanged.
Cairns is a low-lying city and tourist center, with a population of about 100,000
that is growing at about 3% yr-1. Under present conditions, McInnes et al. (2000)
found that the 1-in-100-year event is about 2.3 m in height; under the enhanced
greenhouse conditions, it would increase to about 2.6 m, and with an additional
10- to 40-cm sea-level rise, the 1-in-100-year event would be about 2.7-3.0
m (see Figure 12-4). This would imply greatly increased inundation and wave
damage in such an event, suggesting a possible need for changes in zoning, building
regulations, and evacuation procedures.
Urban areas also are vulnerable to riverine flooding (Smith, 1998a) and flash
floods exacerbated by fast runoff from paved and roofed areas (Abbs and Trinidad,
1996). Considerable effort has gone into methods to improve estimates of extreme
precipitation under present conditions (Abbs and Ryan, 1997; Abbs, 1998). Schreider
et al. (2000) applied a rainfall-runoff model to three different catchments
upstream of Sydney and Canberra under doubled-CO2 conditions. They found increases
in the magnitude and frequency of flood events, but these effects differed widely
between catchments because of the different physical characteristics of each
catchment.
The safety of publicly owned and private dams also is a major issue (ANCOLD,
1986; Webster and Wark, 1987; Pisaniello and McKay, 1998) that is likely to
be exacerbated by increases in rainfall intensity and probable maximum precipitation
(Pearce and Kennedy, 1993; Fowler and Hennessy, 1995; Abbs and Ryan, 1997; Hennessy
et al., 1997).
Vulnerability depends not only on the severity of the potential impacts but
on hazard mitigation measures put in place (including time- and location-specific
hazard prediction), crisis management capability, and policies that avoid or minimize
the hazard. These matters are discussed in Smith (1998a), Handmer (1997), and
Kouzmin and Korac-Kakabadse (1999).
|