12.8.3. Natural Systems
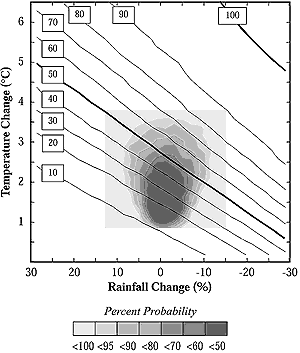
Figure 12-5: Risk response surface incorporating cumulative probability
plots (in shaded box) for climate change magnitudes as indicated on x- and
y-axes. Indicated percentage probabilities are probabilities of climate
change in northern Victoria in 2070 lying within each shaded area (thus,
there is a 100% probability of climate within the shaded square, and a 50%
probability of climate within the innermost region). Probability (in percent)
of irrigation water demand exceeding farm supply cap in any one year, for
indicated climate change, is indicated by oblique lines. Critical threshold
(heavy line) is set at a 50% chance of exceeding the cap (Jones, 2000). |
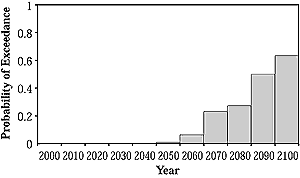
Figure 12-6: Change in probability of exceeding critical threshold
(exceeding farm cap in 50% of all years) over time. Note that if farm cap
were reduced through reductions in irrigation supply under climate change,
the probability of the cap being exceeded would be increased (Jones, 2000). |
A large fraction of the region is composed of unmodified or nonintensively
managed ecosystems where adaptation will depend mostly on natural processes.
Vulnerability will occur when the magnitude or rate of climate variations lies
outside the range of past variations. In some cases, adaptation processes may
be very accommodating, whereas in others adaptation may be very limited. Ecosystems
in the region handle a wide variety of climatic variability, in some cases with
very large swings, but generally this variation occurs on short time scalesup
to a few years. This does not necessarily confer adaptability to long-term changes
of similar magnitude.
An important vulnerability identified by Basher et al. (1998) is the
problem of temperatures in low to mid-latitudes that reach levels never before
experienced and exceed the available tolerances of plants and animals, with
no options for migration. The southwest of western Australia is a case in point
(see Section 12.4.2). Another potential vulnerability
arises from changes in the frequency of events. Examples include a climatic
swing of duration exceeding a reproductive requirement (e.g., water birds in
ephemeral lakesHassall and Associates et al., 1998) and damaging
events occurring too frequently to allow young organisms to mature and ecosystems
to become reestablished.
Vulnerability also is expected to exist where species or ecosystems already
are stressed or marginal, such as with threatened species; remnant vegetation;
significantly modified systems; ecosystems already invaded by exotic organisms;
and areas where physical characteristics set constraints, such as atolls, low-lying
islands, and mountain tops. Coral reefs, for instance, may be able to survive
short periods of rising sea level in clear water but are less likely to do so
in turbid or polluted water or if their growth rates are reduced by acidification
of the ocean (Pittock, 1999). Vulnerability of coastal freshwater wetlands in
northern Australia to salinization resulting from increasing sea level and the
inability of some of these wetlands to migrate upstream because of physical
barriers in the landscape is described in Sections 12.4.5
and 12.4.7.
Unfortunately, there is relatively little specific information about the long-term
capacity for and rates of adaptation of ecosystems in Australia and New Zealand
that can be used to predict likely outcomes for the region. Therefore, a large
degree of uncertainty inevitably exists about the future of the region's
natural ecosystems under climate change.
12.8.4. Managed Systems
In the region's agriculture, many farming systems respond rapidly to external
changes in markets and technology, through changes in cultivars, crops, or farm
systems. Mid-latitude regions with adequate water supplies have many options
available for adaptation to climate change, in terms of crop types and animal
production systems drawn from other climatic zones. However, at low latitudes,
where temperatures increasingly will lie outside past bounds, there will be
no pool of new plant or animal options to draw from, and the productivity of
available systems is likely to decline.
Adaptation options will be more limited where a climatic element is marginal,
such as low rainfall, or where physical circumstances dictate, such as restricted
soil types. Even where adaptations are possible, they may be feasible only in
response to short-term or small variations. At some point, a need may arise
for major and costly reconfiguration, such as a shift from or to irrigation
or in farming activity. Indeed, one adaptation process already being implemented
in Australia to cope with existing competition for water is water pricing and
trading, which is likely to lead to considerable restructuring of rural industries
(see Section 12.4.6).
Management of climate variability in Australia currently involves government
subsidies in the form of drought or flood relief when a specific level of extreme
that is classified as exceptional occurs (Stafford Smith and McKeon, 1998; see
also Section 12.5.6). An adaptive measure being applied
in Australia and New Zealand is to improve seasonal forecasting and to help
farmers optimize their management strategies (Stone and McKeon, 1992; Stone
et al., 1996a; White, 2000), including reducing farm inputs in potentially
poor years.
However, if a trend toward more frequent extremes were to occur, such measures
might not allow farmers to make viable long-term incomes because there may be
fewer good years. The question arises whether this is merely a string of coincidental
extremes, for which assistance is appropriate, or whether it is part of an ongoing
trend resulting from climate change. The alternative policy response to the
latter possibility may well be to contribute to restructuring of the industry.
The PMSEIC (1999) report notes that there are opportunities for new sustainable
production systems that simultaneously contribute to mitigation objectives through
retention of vegetation and introduction of deeper rooted perennial pasture.
Tree farming in the context of a carbon-trading scheme would provide additional
opportunities, and this strategy could be linked to sustainability and alleviation
of dryland salinity.
Nevertheless, the report recognizes that totally new production systems may
be required for sustainability. These systems will need to capture water and
nutrients that otherwise would pass the root zone and cause degradation problems.
The design of such systems will entail research into rotating and mixing configurations
of plants; manipulating phenology; modifying current crops and pastures through
plant breeding, including molecular genetics; and possibly commercializing wildlife
species and endemic biological resources. The report concedes, however, that
there probably still will be agricultural areas where attempts to restore environmental
and economic health will meet with little success.
Vulnerability and the potential for adaptation can be investigated quantitatively
if a system and the climate change impacts on that system can be modeled. This
was done by Pittock and Jones (2000) and Jones (2000) for climatically induced
changes in irrigation water demand on a farm in northern Victoria. Here a window
of opportunity is opened for adaptation via identification of a future time
when adaptation would be necessary to reduce the risk of demand exceeding irrigation
supply.
Jones (2000) used a model that was based on historical irrigation practice.
Seasonal water use was used to estimate an annual farm cap of 12 Ml ha-1,
based on the annual allocated water right. Water demand in excess of this farm
cap in 50% of the years was taken to represent a critical threshold beyond which
the farmer cannot adapt. Conditional probabilities within projected ranges of
regional rainfall and temperature change were utilized, combined with a sensitivity
analysis, to construct risk response surfaces (see Figure
12-5). Monte Carlo sampling was used to calculate the probability of the
annual farm cap being exceeded across ranges of temperature and rainfall change
projected at intervals from 2000 to 2100. Some degree of adaptation was indicated
as desirable by 2030, although the theoretical critical threshold was not approached
until 2050, becoming probable by 2090 (see Figure 12-6).
In a full analysis, this example would need to be combined with an analysis of
the likelihood of changes in water supply (e.g., Schreider et al., 1997)
affecting the allocated irrigation cap and an evaluation of possible adaptation
measures.
|