13.1.3. Recent Climate Variability in Europe, including Recent Warming
The climate of Europe exhibits large differences from west (maritime) to east
(continental) and from north (Arctic) to south (Mediterranean). The climatic
effects of the distribution of land and ocean are further complicated by numerous
high mountain ranges, which act as physical barriers to atmospheric circulation
and often introduce large precipitation gradients within small regions (e.g.,
Frei and Schär, 1998). There is no synoptic consistency to the behavior of the
European atmosphere across such a heterogeneous climatic domain.
Europe possesses long instrumental data records. The central England temperature
series, for example, commences in 1659 (Jones and Hulme, 1997). Reconstruction
of regional climates in Europe with proxy data sets identifies the magnitudes
of natural temperature variability that have occurred on even longer multi-century
time scales: 1,400 years of summer temperatures from tree growth in Fennoscandia
(Briffa et al., 1990), 460 years of monthly temperature and precipitation patterns
from hydrological and biological evidence in central Europe (Pfister, 1992),
and 500 years of annual temperatures from ice cores in Greenland (Fischer et
al., 1998). Direct sea-level measurements are available from the 18th century.
There have been several attempts at deciphering the remote past, based on proxy
data (e.g., Velichko et al., 1998; Zelikson et al., 1998).
13.1.3.1. Temperature
Most of Europe has experienced increases in surface air temperature during
the 20th century that, averaged across the continent, amount to about 0.8°C
in annual temperature (see Figure 13-1) (ECSN, 1995; Beniston
et al., 1998; EEA, 1998). This warming has been largest over northwestern Russia
and the Iberian peninsula (Nicholls et al., 1996; Onate and Pou, 1996) and stronger
in winter than in summer (Maugeri and Nanni, 1998; Brunetti et al., 2000). An
exception is Fennoscandia, which has recorded cooling in mean maximum and mean
minimum temperature during 1910–1995 in winter but warming in summer (Tuomenvirta
et al., 1998). The past decade in Europe (1990–1999) has been the warmest in
the instrumental record, annually and for winter. Increases in growing-season
length also have been observed in Europe—for example, in western Russia (Jones
and Briffa, 1995) and in Fennoscandia (Carter, 1998). Trends in the intensity
of the growing season as measured by growing degree days, however, are more
ambiguous in both of these studies. The evidence for longer growing seasons
in Europe also is supported by phenological data collected in central Europe
(Menzel and Fabian, 1999). These data point to increases of about 10 days in
average growing-season length since the early 1960s. Other biological indicators
of a changing growing season in Europe include poleward shifts of 35–240 km
during this century in entire ranges of 34 different butterfly species (Parmesan
et al., 1999) and earlier breeding times for several species of amphibians and
migratory birds during the past few decades (Forchhammer et al., 1997).
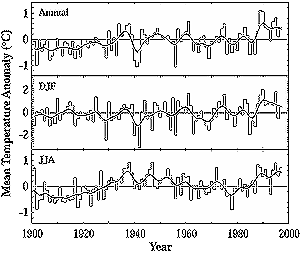
Figure 13-1: European annual mean temperature anomalies over land, 1901–1995,
for the region 35°N to 75°N and 30°W to 50°E, with respect to 1961–90 mean.
10-pt filter applied. Data from IPCC Data Distribution Centre. |
Warming in annual mean temperature has occurred preferentially
as a result of nighttime rather than daytime temperature increases (Brazdil et
al., 1996; Easterling et al., 1997; Tuomenvirta et al., 1998), reflecting similar
tendencies to those in other world regions. There has been some evidence that
this reduction in the diurnal temperature range (DTR) has been associated with
increased cloudiness. This is especially true over parts of the former Soviet
Union (FSU—Abakumova et al., 1996; Groisman et al., 1996), Fennoscandia (Kaas
and Frich, 1995; Tuomenvirta et al., 1998), and Switzerland, where Rebetez and
Beniston (1998) found that a 20th-century decrease in DTR of about 1.5°C was strongly
correlated with increased cloudiness, except at high elevations. In Italy (Brunetti
et al., 2000), daytime warming is higher than nighttime temperature rise, with
a consequent increase in DTR.
13.1.3.2. Precipitation
Trends in annual precipitation differ between northern Europe (wetting) and
southern Europe (drying), reflecting a wider hemispheric pattern of contrasting
zonal-mean precipitation trends between high and low latitudes (Dai et al.,
1997; Hulme et al., 1998). Precipitation over northern Europe has increased
by 10–40% in the 20th century, whereas some parts of southern Europe have dried
by as much as 20% (see Figure 13-2). Romero et al. (1999)
show that the numbers of days with precipitation over the Spanish southern coast
and the Pyrenees region have decreased by 50 and 30%, respectively, in 1964–1993.
In Italy, total precipitation in the 20th century has decreased by about 5%
in the north and by about 15% in the south (Buffoni et al., 1999; Brunetti et
al., 2001). Analysis of moisture extremes over Europe, using the Palmer Drought
Severity Index (PDSI—Briffa et al., 1994) showed strong decadal-scale variability
in drought frequency; the 1940s and early 1950s experienced widespread and severe
droughts—a pattern repeated in 1989 and 1990.
13.1.3.3. Extreme Events
Analyses of trends in extreme weather events in Europe generally have been
limited to national studies, making it difficult to provide a continent-wide
overview of changes in hot/cold day frequencies, precipitation intensities,
or gale frequencies. Gruza et al. (1999) analyzed data over the whole of Russia,
the western third of which falls into our definition of Europe, and found a
slight increase over the 20th century in the Climate Extremes Index (CEI), which
combines daily temperature, daily precipitation, and drought extremes. Analysis
of 85 long-term maximum 1-day precipitation records in the Nordic countries
indicates that there is a maximum in the 1930s and a tendency of increasing
values during the 1980s and 1990s—decades with relatively high regional summer
temperatures. In western Norway, the past 2 decades have been exceptional, with
substantial increases in orographic precipitation during autumn, winter, and
spring (Førland et al., 1998). Elsewhere, daily precipitation intensities over
the UK have increased in winter over recent decades (Osborn et al., 1999), although
not in other seasons. This increase in UK winter precipitation intensities has
been paralleled by a marked decrease in the frequency of cold winter days in
the UK (Jones et al., 1999). Changes in storminess over the northeast Atlantic
have been analyzed by Schmidt et al. (1998) and WASA (1998); they show that
although storminess has increased in recent decades, storm intensities are no
higher than they were early in the 20th century. Wave heights around the shores
of northwest Europe also show large decadal variability, but no long-term trends
emerge.
13.1.3.4. North Atlantic Oscillation
One important cause for interannual and perhaps interdecadal climate variability
in Europe, particularly in winter, is the North Atlantic Oscillation (NAO).
NAO is a measure of the strength of the westerly flow over the North Atlantic;
records go back to the early 19th century (Jones et al., 1997). Over the past
4 decades, the NAO pattern gradually has altered from the most extreme and persistent
negative phase in the 1960s to the most extreme positive phase during the late
1980s and early 1990s—a trend that has been responsible for relatively mild
and wet winters during the latter period over much of northwest Europe (Hurrell
and van Loon, 1997) and can help explain the observed narrowing in DTR (Tuomenvirta
et al., 1998). Following its long period of amplification, the NAO index underwent
a sharp decrease to a short-lived minimum in the winter of 1995–1996, with radical
recognizable changes in the North Atlantic. It should be noted that the NAO
appears to exert significant control on the export of ice and freshwater from
the Arctic to the open Atlantic (WCRP, 1998). Since that temporary minimum,
a recovery toward positive values of the NAO has been observed. NAO certainly
is a more important influence on European climate than the El Niño-Southern
Oscillation (ENSO), although several studies have explored the influence of
ENSO on European precipitation variability, and links between NAO and ENSO are
being sought. Evidence for such an influence is weak and shows phase differences
through the continent (Fraedrich and Müller, 1992; Fraedrich et al., 1992; Rodo
et al., 1997; Price et al., 1998).
13.1.4. Key Sensitivities to Climate and Weather Now
The response of human activities and the natural environment to current weather
perturbations provides a guide to where critical sensitivities to future climate
change may lie. Even if adaptation may modify the response, analysis of present-day
sensitivities helps us understand the likely impact of future climate changes
on our environment and lifestyle.
The principal sensitivities in Europe to current climate and weather conditions
are to:
- Extreme seasons – Exceptionally hot, dry summers – Mild winters
- Short-duration hazards – Windstorm (possibly associated with tidal surges)
– Heavy rain leading to river-valley flooding and flash floods
- Slow, long-term change – Coastal squeeze.
Sensitivities vary across Europe as a result of differences in climate and
topography, as well as the socioeconomic environment, but all of Europe is sensitive
to the foregoing anomalous conditions.
13.1.4.1. Extreme Seasons
13.1.4.1.1. Exceptionally hot, dry summers
Extremely hot and dry summers were experienced in 1995 throughout much of western
Europe (Palutikof et al., 1997) and in 1992 in CEE (Schellnhuber et al., 1994).
Summer drought conditions can have a devastating impact on the natural environment,
particularly by reducing the availability of water for flora and fauna. Pollution
levels may rise to high levels in the subsiding anticyclonic air, with add-on
effects for human health in terms of increased numbers of asthma attacks and
associated hospitalizations. High water temperatures often lead to algal blooms,
which may make water bodies unusable for recreational purposes.
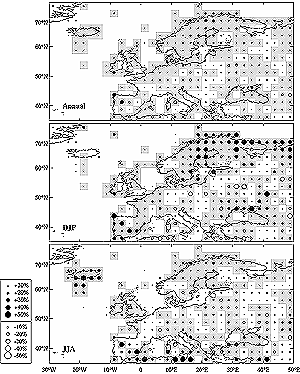
Figure 13-2: Trends in annual (top), winter (middle), and summer (bottom)
precipitation expressed as % per century and calculated on 2.5° grid. Black
circles denote wetting and white circles denote drying.Magnitude of trend
is related to circle size. Shaded trends are significant at 90% (data from
New et al., 1999). |
Agriculture and water supply are the two economic sectors
that may be most severely affected by exceptional heat and drought in summer.
Large proportions of the working populations in Mediterranean countries are
engaged in agriculture, and even in “normal” years water shortages may be a
limiting factor. These countries, therefore, appear to be more vulnerable to
exceptional summer weather. An increased frequency of hot, dry summers is likely
to reduce tree growth and affect timber yield and quality. Reduced tree vigor
often favors outbreaks of pests and pathogens, and hot and dry conditions trigger
wildfire.
13.1.4.1.2. Mild winters
Milder winters exert a major impact on the natural environment. Overwintering
of species is more successful. However, failure to kill off pests and diseases
that prey on wildlife, as well as failure to cull weaker members of the specieswhich
then compete for food in the following springs and summersin the end may
be counterproductive. Cannell and Pitcairn (1993) show that during two mild
winters in the UK, various life events occurred earlierfor example, insects
and other animals moved about and fed more actively, causing widespread aphid
damage on oak and mite damage on lime. Milder winters can affect fulfillment
of chilling requirements in some plant species, which would have an impact on
the formation of leaf and flower buds (e.g., Murray et al., 1989). This could
lead to regeneration failure; some susceptible species are common across much
of Europe today (e.g., Picea abies) (Sykes and Prentice, 1996).
The skiing industry in Europe is vulnerable to variations in snowfall—that
is, too little or too much snow. Many European ski resorts have suffered severe
shortfalls in earnings when snow cover was not sufficiently deep during Christmas
and winter school holidays. If temperatures remain below freezing, a more active
hydrological cycle might lead to more episodes of heavy snowfall; the winter
of 1998–1999 is a good proxy for this situation. Although the number of cold
spells generally has decreased over the past few decades, cold spells still
have a range of impacts on agriculture, settlements, the built environment,
and transport.
13.1.4.2. Short-Duration Hazards
Severe windstorms affected Europe in the 1990s. The January 1990 storm (Daria)
caused insured losses of about US$5.7 billion (at 1997 prices) and 95 deaths;
in the following month, the storm of 26 February (Vivian) caused a further US$3.9
billion in insured losses (Swiss Re, 1998) and 64 deaths. The UK experienced
a severe windstorm at Christmas 1997, causing 13 deaths and losses of US$500
million (Swiss Re, 1998).
The major impact of windstorm on the natural environment is on trees, woodland,
and forest. It is estimated that 15 million trees (5 months’ production of coniferous
wood and 2 years’ production of broadleaf timber production—see Quine, 1988)
were blown down in the UK during the October 1987 storm.
Windstorm associated with tidal surge is a particularly deadly combination
for low-lying coastal areas, as epitomized by the event of 1953. Severe northerly
winds combined with an exceptionally high spring tide caused overtopping in
The Netherlands and much of East Anglia, resulting in more than 2,000 deaths.
Since then the policy has been to keep the sea out at all costs, and in fact
several storm surges of similar size have occurred with no loss of life.
Several devastating river floods, of high severity, occurred in Europe in the
1990s (Kundzewicz and Takeuchi, 1999). Among the flood events to hit the headlines
was the Odra flood in the summer of 1997, during which historic flow records
were broken. The deluge caused more than 100 deaths and economic damage in excess
of US$5 billion (Kundzewicz et al., 1999).
13.1.4.3. Coastal Squeeze
Coastal squeeze occurs when coastal habitats are “squeezed” between rising
sea level and fixed hard defenses (Bijlsma et al., 1996). It is occurring in
most northwest European countries, and it already is a coastal management issue
(Rigg et al., 1997). It is considered in more detail in Sections
13.2.1.3 and 13.3.5.
|