18.4.3. Evaluation of Adaptation Options and Adaptation Costs
Some very general steps for identifying and evaluating planned adaptations
are given in Carter et al. (1994) and UNEP (1998). Somewhat more detailed
procedures for evaluating anticipatory adaptation policies in the climate change
context are outlined in Smith and Lenhart (1996) and Smith (1997). This approach
addresses management of institutional processes and players and proposes net
benefits and implementability as central evaluative criteria. Numerous other
considerations are noted, including flexibility, benefits independent of climate
change ("no regrets"), local priorities, levels of risk, and time
frames of decisions. From a disaster management perspective, Tol et al.
(1996) argue that policies must be evaluated with respect to economic viability,
environmental sustainability, public acceptability, and behavioral flexibility.
Tol et al. (1999) apply these observations in an examination of adaptation
to increased risk of river floods in The Netherlands. They note several possible
adaptations, but none could be accomplished without creating significant distributional
and/or ecological impacts. None, therefore, would be feasible without enormous
political will and institutional reform. Klein and Tol (1997) and UNEP (1998)
describe methodologies for evaluation, including cost-benefit, cost-effectiveness,
risk-benefit, and multi-criteria methods. Multi-criteria methods to evaluate
possible adaptation options have been demonstrated for coastal zones (El-Raey
et al., 1999) and agriculture (Mizina et al., 1999).
Fankhauser (1996) provides an economic efficiency framework in which adaptation
actions are considered justified as long as the additional costs of adaptation
are lower than the additional benefits from the associated reduced damages.
Optimal levels of adaptation (in an economic efficiency sense) are based on
minimizing the sum of adaptation costs and residual damage costs. Such studies
require the definition of a base case that involves estimation of autonomous
adaptations. These and other normative studies (e.g., Titus, 1990; Goklany,
1995) illustrate the range of principles and methods that have been proposed
for identifying, evaluating, and recommending (planned) adaptation measures.
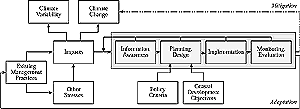
Figure 18-6: Conceptual framework showing, in shaded
area, iterative steps involved in planned coastal adaptation to climate
variability and change (Klein et al., 1999).
|
There are, however, few comprehensive estimates of the costs of adaptation.
Mimura and Harasawa (2000) report estimates of 11.5-20 trillion Yen as
the cost of maintaining the functions of Japanese infrastructure against a 1-m
rise in sea level. Yohe and Schlesinger (1998) applied a cost-benefit rule to
adaptation decisions across a sample of the developed coastline of the United
States. With a 3% discount rate, their national estimates of the expected discounted
cost of protecting or abandoning developed coastal property in response to sea-level
rise that is based on a mean greenhouse emissions scenario is US$1.3 billion
with foresight and US$1.8 billion without. Their estimates climb to more than
US$4 billion and 5 billion, respectively, along the 1-m sea-level rise scenario
that matches the Mimura and Harasawa study. Between 55 and 70% of these costs
were attributed to planned adaptation. The remainder reflect estimates of residual
damage associated with abandoning property with and without completely efficient
autonomous adaptation. Indeed, the differences between the foresight and non-foresight
estimates can be regarded as estimates of the incremental cost of incomplete
autonomous adaptation in advance of planned responses.
On a more local scale, Smith et al. (1998) report cost estimates that
are clearly sensitive to design and evaluation criteria. For example, none of
the five flood protection strategies for the southernmost part of the Dutch
Meuse (assuming 10% more winter precipitation and a warming of 2°C) would
achieve economic benefits that exceed their costs of DGL 243-1,505 million,
given a 5% discount rate. Moreover, only building quays would meet the benefit-cost
standard with a 5% discount rate. Nonetheless, the government chose a wildlife
renovation strategy on the basis of additional benefits for nature and recreation.
Smith et al. (1998) also report that the cost of raising the Northumberland
Bridge between Prince Edward Island and New Brunswick to accommodate a 1-m sea-level
rise would be US$1 billion or 250,000, depending on whether the entire bridge
or only the portion that spanned the shipping lanes were raised.
Klein et al. (1999) develop a conceptual framework of the process of
planned adaptations, aimed at changing existing management practices in coastal
zones. In this model, adaptation is a continuous and iterative cycle, involving
several steps: information collection and awareness raising, planning and design
(incorporating policy criteria and development objectives), implementation,
monitoring, and evaluation (see Figure 18-6).
18.4.4. Public Adaptation Decisions, Uncertainty, and Risk Management
Research increasingly addresses how adaptation is considered in actual policy
decisionmaking. Stakhiv (1996) and Frederick (1997), dealing with the U.S. water
resources sector, conclude that existing institutions and planning processes
can deal with climate change; such processes essentially represent adaptive
management. As in many other sectors and circumstances, adaptation to climate
change hazards in the coastal zone is part of ongoing coastal zone management.
Adaptation to sea-level rise and extreme climate events is being included in
Japanese coastal policies (Mimura and Kawaguchi, 1997), British shoreline management
(Leafe et al., 1998), and Dutch law and coastal zone management (Koster
and Hillen, 1995; Helmer et al., 1996; Klein et al., 1998).
Planning of adaptation invariably is complicated by multiple policy criteria
and interests that may be in conflict (Hareau et al., 1999). For example,
the economically most efficient path to implement an adaptation option might
not be the most effective or equitable one. Moreover, decisions have to be made
in the face of uncertainty (Lempert et al., 2000). Uncertainties that
are pertinent to adaptation are associated with climate change itself, its associated
extremes, their effects, the vulnerability of systems and regions, conditions
that influence vulnerability, and many attributes of adaptations, including
their costs, implementability, consequences, and effectiveness (Campos et
al., 1996; Lansigan et al., 1997; Handmer et al., 1999; Murdiyarso,
2000).
Given these uncertainties, it is not surprising that adaptation strategies
frequently are described as forms of risk management. For example, adaptations
to deal with climate change impacts or risks to human health can be biological
(acquired immunity), individual (risk-aversion options), or social (McMichael
et al., 1996). Most social adaptation strategies are measures to reduce
health risks via public health programs (Patz, 1996; McMichael and Kovats, 2000).
Similarly, public adaptations via "disaster loss mitigation" (Bruce,
1999) are mainly risk management initiatives such as improved warning and preparedness
systems, less vulnerable buildings and infrastructure, risk-averse land-use
planning, and more resilient water supply systems. Nguyen et al. (1998),
Hisschemöller and Olsthoorn (1999), and Perez et al. (1999) also
describe adaptations to climate change and extremes as modifications to existing
risk management programs. As de Loë and Kreutzwiser (2000) and others point
out, it remains unclear whether practices designed for historical climatic variability
will be able to cope with future variability.
To recognize these uncertainties, decision tools to help evaluate adaptation
options include risk-benefit and multi-criteria analyses (Klein and Tol, 1997).
Such evaluations are further complicated by the existence of secondary impacts
related to the adaptation itself. For example, water development projects (adaptations
to water supply risks) can have significant effects on local transmission of
parasitic diseases, including malaria, lymphatic filasiasis, and schistosomiasis
(Hunter et al., 1993; McMichael and Kovats, 2000). Improved water supply
in some rural areas of Asia has resulted in a dramatic increase in Aedes mosquito
breeding sites and, consequently, outbreaks of dengue (WHO, 1997). Langen and
Tol (1999) provide examples of technical response options to climate hazards
that are counterproductive in the longer term. Existing resource management
programs do not necessarily consider changed risks or recognize local interests
and inequities (Primo, 1996). Wilhite's (1996) analysis of programs in
the United States, Australia, and Brazil shows the ineffectiveness of reactive
crisis management approaches and the need for proactive and cooperative planning.
Nonetheless, it is widely accepted that planned adaptations to climate risks
are most likely to be implemented when they are developed as components of (or
as modifications to) existing resource management programs or as part of national
or regional strategies for sustainable development (Campos et al., 1996;
Magalhães, 1996; Theu et al., 1996; Mimura, 1999a; Apuuli et
al., 2000; Munasinghe, 2000; Osokova et al., 2000).
|