4.2 Land Use, Land-Use Change, and Carbon Cycling in Terrestrial
Ecosystems
Terrestrial ecosystems provide an active mechanism (photosynthesis) for biological
removal of CO2 from the atmosphere. They act as reservoirs of photosynthetically-fixed
C by storing it in various forms in plant tissues, in dead organic material,
and in soils. Terrestrial ecosystems also provide a flow of harvestable products
that not only contain carbon but also compete in the market place with fossil
fuels, and with other materials for construction (such as cement), and for other
purposes (such as plastics) that also have implications for the global carbon
cycle (see Box 4.1).
Box 4.1. Stocks and Flows
The global carbon cycle consists of the various stocks of carbon in the
earth system and the flows of carbon between these stocks. It is discussed
at length in IPCC WG I (Prentice et al., 2001) and IPCC Special Report
on LULUCF (IPCC, 2000a) and is illustrated in Figure
4.2.
|
Figure 4.2: Different ecosystems, their components,
and human activities. The carbon stocks associated with the different
ecosystems are stored in aboveground and belowground biomass, detrital
material (dead organic matter), and soils. Carbon is withdrawn from
the atmosphere through photosynthesis (vertical down arrow), and returned
by oxidation processes that include plant respiration, decomposition,
and combustion (vertical up arrow). Carbon is also transferred within
ecosystems and to other locations (horizontal arrows). Both natural
processes and human activities affect carbon flows. Mitigation activities
directed at one ecosystem component generally have additional effects
influencing carbon accumulation in, or loss from, other components.
Estimates of ecosystem and atmospheric C stocks are adapted from Bolin
et al. (2000). Values for C stocks in some ecosystems are still
very uncertain. Not shown are estimates of C stocks in tundra (127GtC),
deserts and semi-deserts (199GtC), and oceans (approx. 39,000GtC)
(numbers are taken from Special Report on LULUCF, Fig 1-1, page 30;
IPCC, 2000a). |
A consequence of the conservation of mass is that the net of all of the
flows (measured as a rate variable in units such as tC/yr) into and out
of a given reservoir or stock (measured in units such as tC) during a
period of time must equal the change in the stock (tC) in that period.
Conversely, a change in stock of a reservoir during a given period must
exactly equal the integrated net difference in C flows into and out of
that reservoir during that period. Elsewhere in this text the word pool
is sometimes used to represent the various reservoirs of carbon in the
global carbon cycle. The word sink is used to indicate the
net positive flow of carbon into a terrestrial carbon pool.
The maximum rate of net ecosystem carbon uptake cannot occur at the same
time as the maximum ecosystem carbon stock (see Figure
4.3). An ecosystem depleted of carbon by past events may have much
higher rates of carbon accumulation than a comparable one in which carbon
stocks have been maintained. Ecosystems eventually approach some maximum
carbon stock a carrying capacity at which time the flows
into the carbon pool are balanced by flows out of the carbon pool. Because
C sink and C stock in ecosystems cannot be maximized simultaneously, mitigation
activities aimed at enhancing the sink and maintaining the biological
carbon stock coincide only partially (IGBP, 1998).
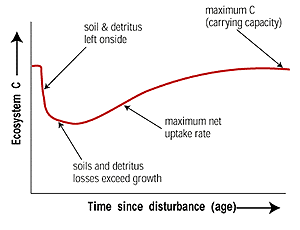 |
Figure 4.3: An example of net changes in ecosystem
carbon stocks over time. Changes in individual ecosystem components
take place at different rates, but it is the net of the changes in
all interconnected pools that determines the net flow to or from the
atmosphere. In the example, the accumulation of biomass initially
is at a lower rate than the decomposition of the dead organic matter
stock so the stock of ecosystem C declines. Later in the cycle, dead
organic matter stocks may increase, although other components have
reached a steady state. Maximum ecosystem stocks (highest value of
ecosystem C) occur at a later time than the maximum rate of net carbon
uptake (steepest slope of the ecosystem C line). |
Similarly, the maximum rate of C substitution cannot occur at the same
time as maximum C conservation. High rates of carbon substitution, through
use of forest products or biofuels, generally require high productivity
and efficient manufacture and use of derived products.
Carbon taken up by the biosphere may also accumulate in offsite pools
as products or in landfills but it continues to oxidize
at rates that depend on the conditions of those pools. It is the net of
many flows that defines the changes in carbon stocks of off-site pools
as well as of on-site pools. Carbon accumulation in off-site pools is
an often overlooked, but a potentially important, form of sequestration.
|
Human activities have changed terrestrial carbon pools. The largest changes
occurred with the conversion of natural ecosystems to arable lands. Such disruptions
typically result in a large reduction of vegetation biomass and a loss of about
30% of the C in the surface 1 metre of soil (Davidson and Ackermann, 1993; Anderson,
1995; Houghton, 1995a; Kolchugina et al., 1995). Globally, conversion to arable
agriculture has resulted in soil C losses of about 50GtC (Harrison et al., 1993;
Scharpenseel and Becker-Heidmann, 1994; Houghton, 1995a; Cole et al., 1996;
Paustian et al., 2000), and total emissions of C from land use change, including
that from biomass loss, have amounted to about 122 ± 40GtC (Houghton,
1995b; Schimel, 1995). Most of the soil C losses occur within a few years or
decades of conversion, so that in temperate zones, where there is little expansion
of agricultural lands now, losses of C have largely abated (Cole et al., 1993;
Anderson, 1995; Janzen et al., 1998; Larionova et al., 1998). Tropical areas,
however, remain an important source of CO2 because of widespread
clearing of new lands and reduced duration of fallow periods in
shifting agriculture systems (Paustian et al., 1997b; Scholes and van Breemen,
1997; Woomer et al., 1997; Mosier, 1998).
The competition for land varies among countries and within a country. Land-use
and forestry policies for C management may be most successful when climate mitigation
is considered alongside other needs for land, including agriculture, forestry,
agroforestry, biodiversity, soil and water conservation, and recreation. Forest
fires, for example, are controlled, in many parts of the world, not as a measure
for carbon mitigation, but simply because fire threatens areas of human settlement
and the habitats of living organisms.
Similarly, biodiversity and landscape considerations have motivated protection
of old-growth stands in temperate, boreal, and tropical rain forests from commercial
logging. In many cases such decisions have prevented C release into the atmosphere,
even though C mitigation was not the initial intent (Harmon et al., 1990). The
impact of harvest restrictions on C pool in old-growth forests may be affected
by leakage. If one ecosystem is protected but timber demand remains
constant, logging may simply be shifted to another, similar ecosystem elsewhere,
perhaps to a country where conservation priorities are lower.
|