A3.1 Introduction
Alternatives and substitutes for HFCs, perfluorocarbons (PFCs), and ozone depleting
substances (ODSs) have recently been extensively evaluated. The Montreal Protocol
Technology and Economic Assessment Panel (TEAP) and its technical committees
published a comprehensive assessment (UNEP, 1999b). Furthermore, reports were
published within the framework of the joint IPCC/TEAP workshop (IPCC/TEAP, 1999)
and the second non-CO2 greenhouse gases conference (van Ham et al.,
2000).
The HFCs that are projected for large volume use have global warming potentials
(GWPs) which are generally lower than those of the ODSs they replace. The GWP
of HFCs replacing ODSs range from 140 to 11,700. HFC-23 with a GWP of 11,700
is used as a replacement for ODSs to only a very minor extent. However, there
are relatively large emissions of HFC-23 from the HCFC-22 manufacturing process.
The majority of HFCs have GWPs much lower than that of HFC-23. PFCs have GWPs
that are generally higher than those of the ODSs they replace, ranging from
7,000 to 9,200 (IPCC, 1996). Table A3.1 lists the atmospheric
properties of the HFCs and HFC blends considered in this Appendix.
Table A3.1: Atmospheric properties (lifetime,
global warming potential (GWP)) for the HFC chemicals described in the Appendix
(IPCC, 1996; WMO, 1999) |
 |
Sub-sector |
Chemical
formula |
Lifetime (yr)
(IPCC, 1996)
|
GWP (100 yr)
(IPCC, 1996)
|
Lifetime (yr)
(IPCC, 2000)
|
GWP (100 yr)
(IPCC, 2000)
|
 |
HFC-23
HFC-32
HFC-125
HFC-134a
HFC-143a
HFC-152a
HFC-227ea
HFC-245faa
HFC-365mfca
HFC-43-10mee |
CHF3
CH2F2
CHF2CF3
CH2FCF3
CH3CF3
CF3CHFCF3
CF3CH2CHF2
CF3CH2CF2CH3
CF3CHFCHFCF2CF3
|
264
5.6
32.6
14.6
48.3
1.5
36.5
-
-
17.1
|
11,700
650
2,800
1,300
3,800
140
2,900
-
-
1,300
|
260
5.0
29
13.8
52
1.4
33
7.2
9.9
15
|
12,000
550
3,400
1,300
4,300
120
3,500
950
890
1,500
|
|
|
|
|
|
|
R-404A
(44% HFC-125,
4% HFC-134a,
52% HFC-143a)
R-407C
(23% HFC-32,
25% HFC-125,
52% HFC-134a)
R-410A
(50% HFC-32,
50% HFC-125)
R-507
(50% HFC-125,
50% HFC-143a) |
|
|
|
|
|
 |
Most HFCs are used for energy-consuming applications such as refrigeration,
air conditioning and heat pumps, and building and appliance insulation. Life
cycle climate performance (LCCP) analysis is being used to estimate the net
contribution to climate change. It includes all direct greenhouse gas emissions
and indirect emissions related to energy consumption associated with the design
and the operational modes of systems (UNEP, 1999b; Papasavva and Moomaw, 1998).
The LCCP is a very system specific parameter that can be used to make relative
rankings. However, LCCP analysis involves regional differences including
different fuel sources and the related equipment operating conditions;
the results can therefore not be generalized in order to make globally valid
comparisons.
The energy efficiency of equipment and products can be expressed in at least
three ways: theoretical maximum efficiency, maximum efficiency achievable with
current technology, and actual efficiency for commercial scale production (often
expressed as a range of values). Systems optimized for a new refrigerant have
been compared to sub-optimum systems with other refrigerants. Furthermore, appliance
sizes and features that influence energy performance vary between studies and
test conditions, and methodologies are often significantly different. These
factors have led to a wide range of energy efficiency claims in technical reports
and commercial publications. Ultimately, the performance and cost effectiveness
of specific products from commercial scale production must be directly compared.
Furthermore, costs reported in this appendix might not always be comparable
because of differing estimation methods, including estimates based on both consumer
and producer costs.
A3.1.1 Past Trends
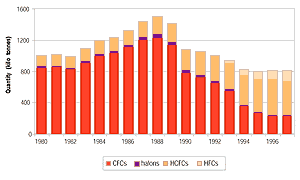
Figure A3.1: Estimated global consumption of CFCs, halons, HCFCs,
and HFCs (McFarland, 1999).
|
Unlike anthropogenic greenhouse gases emitted as an immediate consequence of
the burning of fossil fuels to generate energy, most HFCs and PFCs are contained
within equipment or products for periods ranging from a few months (e.g., in
aerosol propellants) to years (e.g., in refrigeration equipment) to decades
(e.g., in insulating foams). Thus, emissions significantly lag consumption and,
because HFC systems are relatively new, emissions will continue to grow after
2010.
Both the quantities used and patterns of use of ODSs, HFCs, and PFCs are changing
(see Figure A3.1) as ODSs are phased out under the Montreal
Protocol (IPCC/TEAP, 1999; McFarland, 1999). In 1986, less than half of total
ODS use was in insulating foams, fire protection, refrigeration, air conditioning,
and heat pumps, with more than half as aerosol product propellants, non-insulating
foam, solvent, and specialized applications. However, by 1997, the global consumption
of fluorocarbons (CFCs, HCFCs and HFCs) had decreased by about 50% as solvent,
aerosol product, and non-insulating foam applications switched to alternatives
other than fluorocarbons. Refrigeration, air conditioning, and insulating foam
accounted for about 85% of the remaining total fluorocarbon use. 80% of projected
chlorofluorocarbon demand was avoided by reducing emissions, redesign, and use
of non-fluorocarbon technologies. As CFCs, halons, and HCFCs are phased out
globally, the quantities of fluorocarbons are expected to continue to decline
in the short term, but are expected to grow in the longer term.
A3.1.2 Projections
Future global HFC and PFC consumption and/or emissions as substitutes for ODSs
have been separately estimated by IPCC (1995), Midgley and McCulloch (1999),
and UNEP (1998a). Midgley and McCulloch (1999) projected carbon-equivalent emissions
of HFCs and PFCs (excluding unintended chemical by-product emissions) at 60MtCeq
in 2000, 150MtCeq in 2010 and 280MtCeq in 2020. Projected
consumption data for 2000 and 2010 are primarily based on UNEP reports (UNEP,
1998f, 1999b) and are shown in Table A3.2. Considering
that emissions lag consumption by many years, the Midgley and McCulloch figures
are much larger than the UNEP figures. This discrepancy is consistent with the
Midgley and McCulloch scenario which was constructed to represent plausible
upper limits to future emissions (McFarland, 1999). HFC emissions in the SRES
scenarios (IPCC, 2000) are 54MtCeq in 2000 and 130-136MtCeq
in 2010. These values are higher than those presented in Table
A3.2 because of the top-down approach used in SRES that does not adequately
account for delay between use and emissions in the 2000 to 2010 timeframe. Considering
this fact and given the options for substitution, containment, etc., it is estimated
that emissions in 2010 could well be about 100MtCeq below the SRES
forecast at a marginal cost lower than US$200/tCeq. None of the scenarios
have considered the implications of new uses of HFCs or PFCs other than as substitutes
for ODSs.
Projected consumption and emission estimates for HFCs by sub-sector for 2000
and 2010 are summarized in Table A3.2.
|