5.3.1.3. B1 Scenario Family
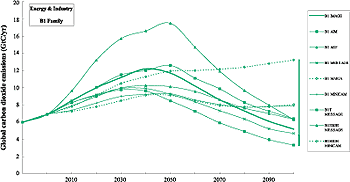
Figure 5-3c: Global CO2 emissions from fossil
fuels and industry in the B1 scenario family (standardized). The marker
scenario is shown with a thick line without ticks, the globally harmonized
scenarios with thin lines, and the non-harmonized scenarios with thin,
dotted lines (see Table 4-3).
|
The strong trend toward ecologically more compatible consumption and production
patterns in the B1 family is reflected by structural changes toward less energy-
and material-intensive activities, which lead to a partial de-coupling of welfare
and energy demands. In the B1 marker scenario (B1-IMAGE; de Vries et al., 2000)
the rapid technological change toward resource saving and ecologically sound
solutions is assumed to spread very quickly, facilitated by high capital stock
turnover rates in currently less developed regions. As a result, energy requirements
in B1-IMAGE increase slowly and a shift away from fossil fuels eventually breaks
the already slow upward trend in carbon emissions (Figure
5-3c). Emissions peak around 2040 at 12 GtC, twice the 1990 level, and by
2100 the emissions fall below the base-year level to 5 GtC. Total cumulative
carbon emissions in the B1-IMAGE scenario amount to 983 GtC by 2100. As for
A1, the population projection adopted for this scenario family declines after
2050.
Rates of energy-intensity improvement in the first half of the 21st century
range quite widely in B1 family scenarios, and lead to emission levels from
8.5 (B1T-MESSAGE) to 17.5 (B1- ASF) GtC in 2050. By 2100 the gap in annual emissions
narrows again, with final emissions between 3 and 8 GtC in all the B1 scenarios
(except B1-High-MiniCAM).
The B1 family also includes one scenario in which energy-related emissions
continue to increase throughout the modeling period, B1-High-MiniCAM. In this
scenario the final energy demand is assumed to rise more rapidly with increasing
income than in the rest of the B1 scenarios. (The total carbon emissions in
B1High-MiniCAM decline slightly later in the modeling period because of reduced
land under management and associated carbon sequestration.)
Total cumulative carbon emissions in the B1 scenario group ranges between 770
and 1390 GtC by 2100. All but two B1 scenarios fall in the low cumulative emissions
category (Table 5-2).
5.3.1.4. B2 Scenario Family
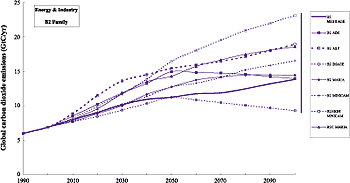
Figure 5-3d: Global CO2 emissions from fossil
fuels and industry in the B2 scenario family (standardized). The marker
scenario is shown with a thick line without ticks, the globally harmonized
scenarios with thin lines, and the non-harmonized scenarios with thin,
dotted lines (see Table 4-3).
|
In the B2 world, dynamics of technological change continue along historical
trends ("dynamics as usual"). The exploitation of comparative regional advantages
in energy resources and technologies leads to regionally different mixes of
clean fossil and non-fossil supply. With the continued growth of population
and of income per capita, a steady increase of CO2 emissions emerges in the
B2 marker (Riahi and Roehrl, 2000), developed using the MESSAGE model (B2-MESSAGE).
By 2050 emissions reach 11 GtC and by 2100 they reach 14 GtC (Figure 5-3d).
Total cumulative CO2 emissions in the B2 marker scenario amount to 1160 GtC
by 2100.
Emissions in the B2 scenarios with harmonized global input assumptions (population,
GDP, final energy; B2-MESSAGE, B2-AIM, B2-MARIA) are very close in 2100. Differences
in emissions are largest around 2050, which reflects the different patterns
of structural change in the energy systems in anticipation of depletion of conventional
oil and gas. The emissions range for non-harmonized scenarios in the B2 scenario
group is larger - between 11.2 (B2-IMAGE6)
and 15.4 (B2-ASF) GtC by 2050. Relatively high emissions in the B2- ASF scenario
are explained by a large share of coal in the fuel mix, because of high oil
and gas prices. In this scenario, coal is also widely used for synthetic liquid
and gaseous fuel production. In 2100, B2-IMAGE emissions (9 GtC) drop below
the B2 marker level, while in B2-ASF emissions continue to grow and reach 19
GtC by 2100. The B2 high scenario variant developed using MiniCAM assumes far
less efficiency gains, smaller available resources of oil and gas, and less
favorable development of solar power costs than in the B2 marker. As in B2-ASF,
the near exhaustion of oil supplies in B2High-MiniCAM leads to a heavy reliance
on synthetic fuels to supply the needs of the transportation sector. Emissions
in this scenario increase to 23 GtC by 2100. An additional alternative with
more coal use (B2C-MARIA) was explored using the MARIA model. Emissions in this
scenario are considerably higher than in the original B2-MARIA case, but are
very close to those of B2-ASF (19 GtC).
Total cumulative carbon emissions across the B2 scenario group range between
1164 and 1686 GtC by 2100. Three of the eight B2 family scenarios fall into
the "medium-high" cumulative emissions category, and the five others fall into
the "medium-low" category (Table 5-2).
5.3.1.5. Inter-family Comparison
Table 5-2 suggests fairly strong contrasts in the
level of cumulative emissions across the four scenario families (note that estimates
in Table 5-2 also include emissions from land use).
A variety of available energy supply and demand options means that cumulative
emissions in the A1 family span the entire set of emission categories. The A2
family scenarios, characterized by a large population and a relatively carbon-intensive
energy system, fall into either the "high" scenario category or in the upper
part of the "medium-high" category. Only the A2-A1-MiniCAM scenario falls in
the "medium-low" category, because of low cumulative emissions prior to 2050.
As a consequence of the low energy consumption and non-fossil energy systems
associated with its sustainable development theme, the B1 family scenarios are
concentrated in the "low" emissions category. Finally, representatives of the
B2 family are present in the two middle categories.
In addition to their cumulative emissions, the scenario families are characterized
by very different emissions trajectories. The A1 fossil fuel scenarios have
continuously increasing emissions, with rapid growth before 2050 and slower
growth thereafter. The A1 scenarios with the "balanced" energy mix (e.g. the
marker A1B-AIM) typically have emissions that decline after 2050, while the
A1T technology scenarios have slower growth prior to 2050, and a steeper decline
after 2050. As for the A1 fossil fuel scenarios, A2 family scenarios are characterized
by high rates of growth in emissions prior to 2050 and subsequently continued
growth but at lower rates. Unlike the rest of the A2 scenarios, the A2-A1-MiniCAM
has more rapid growth in emissions after 2050 than before 2050, because per
capita incomes in a number of developing regions do not reach a level at which
per capita energy demands rise rapidly until the middle of the 21st century.
The B1 family is characterized by lower growth in emissions prior to 2050, mostly
because of lower rates of growth in energy demand, followed by declining emissions
after 2050. Finally, the B2 family is characterized by relatively stable emissions
post-2050, after roughly doubling emissions between 1990 and 2050.
5.3.2. Land-Use Carbon Dioxide Emissions
Most changes in land use are induced by the demand for cropland and grassland,
which is driven by the demand for food products, the extent of biomass energy
use, and policies and practices associated with forest management. The 1990
land-use CO2 emissions remain fairly uncertain, estimated at 1.6 ± 1.0 GtC (Watson
et al., 1996a); a similar level of uncertainty is attached to current land-use
emissions. This uncertainty is reflected by the quantification of the SRES storylines
- the 1990 emission estimates from different models range between 1.0 and 1.6
GtC, while the spread of estimates at the four-region level is even larger.
For the sake of comparability, common, standardized (see Box
5-1 on standardization) emissions are established at 1.1 GtC in 1990 and
1.0 GtC in 2000, to reflect the net carbon flux from contemporary changes in
forest cover.
Generally, the SRES models use different approaches to estimate land-use change
emissions - in some cases the only source of emissions is tropical deforestation,
while in other cases more sources and sinks (including natural) are included7
Moreover, methodologic differences and uncertainties in carbon content, carbon
cycling, and land classification result in seemingly inconsistent results between
models that cover the same land-use sources. These features complicate a straightforward
comparison between land-use emissions in scenarios generated by different SRES
models.
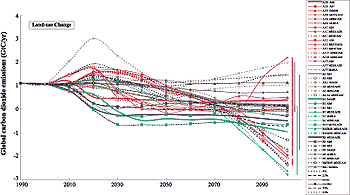
Figure 5-4: Standardized global CO2 emissions
from land-use changes (net balance between anthropogenic changes in sources
and sinks) for 40 SRES scenarios, classified into the four scenario families
(each denoted by a different color code - A1, red; A2, brown; B1, green;
B2, blue). Marker scenarios are shown with thick lines without ticks,
globally harmonized scenarios with thin lines, and non-harmonized scenarios
with thin, dotted lines (see Table 4-3). Black lines show percentiles,
means, and medians for the 40 SRES scenarios. For numbers on the two additional
illustrative scenarios A1FI and A1T see Appendix
VII.
|
Future trajectories of land-use CO2 emissions are shown in Figure 5-4. Emissions
in the 40 SRES scenarios range widely in the same year and change significantly
over time. In scenarios with continued deforestation, emissions rise initially,
then reach a maximum, and finally decline with depletion of forestland that
can be cleared. At the other end of the scenario spectrum, emissions turn negative
and land-use changes become an increasing CO2 sink through afforestation. By
2020, the resultant uncertainty ranges between 0 and 3 GtC across all 40 SRES
scenarios with a median of 1.1 GtC. By 2050, the scenario range shifts to between
-0.7 and 1.2 GtC (median, 0.5 GtC). By 2100 the scenario range lies between
-2.8 and 2.2 GtC, with a median of 0.0 GtC. Interestingly, in specific years
(e.g., in 2050) scenarios from all four SRES families fall within a relatively
narrow emissions corridor (i.e. at least one scenario from each of the four
SRES scenario families falls within the 25th and 75th percentiles of the emission
range). This indicates that similar levels of carbon fluxes related to land
use could arise from widely different socio-economic driving forces, depending
on future trends in food demand and dietary patterns, agricultural productivity
growth, forest practices, etc.
In general, the SRES CO2 land-use emissions follow the same pattern as found
in the literature (see Chapter 3) - initially emissions
increase because of continuing deforestation in developing regions and subsequently
they decrease following a drop in population growth and increases in agricultural
productivity. The main difference between the SRES scenarios and the literature
reviewed in Chapter 3 lies in the maximum emission values,
which are significantly lower in the SRES scenarios. Possibly this arises because
the SRES models explicitly simulate land-use change as a function of pressure
on the land (itself a function of agricultural productivity), while in the literature
land-use CO2 emission scenarios are often based on trend extrapolation or statistical
relationships (e.g. with population growth). The rapid economic development
and technological advances assumed in the SRES storylines thus tend to mitigate
CO2 emissions from deforestation, and in some cases lead to their reversal (e.g.,
turning deforested lands into carbon sinks).
As suggested by Figure 5-4, no simple relation exists between the scenario
families and land-use emissions. As in the case of energy-related emissions,
the A1 family scenarios cover the widest range of emissions and trajectories.
In most cases, emissions in these scenarios decline after 2030 to zero or negative
(carbon sinks) values. However, in two scenarios (A1B-IMAGE and A1B-MARIA),
emissions increase later in the 21st century, reflecting assumptions on additional
land pressures. As a rule, scenarios of the A2 family follow the same convex
trajectory as A1 scenarios, also with two exceptions (A2-IMAGE and A2-AIM).
In accordance with the sustainability emphasis of the B1 family storyline, scenarios
from this family have the lowest initial emissions, which by 2080 or earlier
drop to zero or negative levels. The B1 family marker scenario (B1-IMAGE) has
negative land-use emissions for most of the modeling period, a property directly
related to B1's "environmental conservation" emphasis.
5.3.3. Overview of Sectoral Carbon Dioxide Emissions
As a result of different model specifications and detail, it is not possible
to draw up consistent comparisons between sectoral emissions across different
models. An overview of sectoral CO2 emissions by sector and source category
is summarized in Box 5-2 on the basis of the results
of the MESSAGE (for energy and industrial sources) and AIM (for land-use change
sources) models.
Box 5-2: CO2 Emissions by Sector and Source for
MESSAGE Scenarios
Table 5-4 gives an overview of CO2 emissions by sector and source category
according to the IPCC reporting format given in Watson et al. (1996b).
The differences in sectoral detail across models mean a consistent comparison
and sectoral CO2 emission balances are only possible within one particular
modeling framework. Table 5-4 presents the scenario results as calculated
with the MESSAGE model for 1990, 2050, and 2100 and for the four scenario
families and their scenario groups. Emissions related to land-use change
were derived from consistent model runs with the AIM model.
As in Watson et al. (1996b), emissions are presented by sector, and emission
categories adopt both supply and demand perspectives for energy-related
CO2 emissions. The supply side CO2 balance accounts emissions at the point
of energy combustion, that is at a coal-fired power plant (electric generation)
or by burning coal in industrial boilers (direct fuel use by industry).
Conversely, the demand side CO2 balance accounts emissions per end-use
category, irrespective of whether emissions originate directly at the
point of end-use or upstream in the energy conversion sector. For example,
for residential and commercial energy uses, CO2 emissions include those
from direct fuel combustion as well as those emissions that originate
from the generation of electricity consumed by the residential and commercial
sectors. Finally, an emissions balance by source category is given, in
which emissions are accounted for at the level of primary energy (solids,
liquids, and gases), again after Watson et al. (1996b). Non-energy emissions
are included in a separate "others" category. Combined, these different
emission balances can serve as data input for subsequent mitigation analyses
at the sectoral level or at that of the entire economy.
Table 5-4: Global CO2 (MtC) emissions by sector and source category for seven scenarios calculated with the MESSAGE model for 1990, 2050, and 2100. In the SPM, A1C and A1G scenarios are merged into one fossil-intensive A1FI scenario group (see also footnote
2). |
|
|
1990
|
2050
|
2100
|
|
|
A1B
|
A2
|
B1
|
B2
|
A1T
|
A1G
|
A1C
|
A1B
|
A2
|
B1
|
B2
|
A1T
|
A1G
|
A1C
|
|
Supply Side |
Energy Supply/Transformation |
|
|
|
|
|
|
|
|
|
|
|
|
|
|
|
Electric generation
|
1773
|
4783
|
4875
|
763
|
2844
|
2192
|
6519
|
5924
|
7541
|
9283
|
207
|
5323
|
293
|
8854
|
11166
|
Synfuels production
|
0
|
1162
|
1613
|
2207
|
929
|
1278
|
2294
|
3958
|
170
|
7245
|
2071
|
2848
|
441
|
3619
|
9788
|
Other conversion*
|
680
|
2277
|
1312
|
999
|
1170
|
1506
|
3012
|
719
|
2901
|
2393
|
735
|
1394
|
807
|
7682
|
622
|
|
Direct Use of Fuels by Sector |
|
|
|
|
|
|
|
|
|
|
|
|
|
|
|
Residential/commercial
|
880
|
2782
|
1618
|
1361
|
1494
|
2362
|
3606
|
2458
|
1402
|
925
|
367
|
973
|
656
|
3085
|
1255
|
Industry
|
1289
|
1540
|
1515
|
843
|
1401
|
845
|
987
|
1165
|
273
|
1601
|
176
|
1166
|
503
|
984
|
631
|
Transportation
|
1310
|
3823
|
2952
|
2669
|
2587
|
3445
|
4513
|
5587
|
1881
|
4524
|
1135
|
1845
|
1577
|
5924
|
8640
|
Feedstocks
|
303
|
912
|
1311
|
490
|
891
|
972
|
766
|
768
|
91
|
1963
|
66
|
398
|
448
|
535
|
422
|
|
Non-Energy Emissions |
|
|
|
|
|
|
|
|
|
|
|
|
|
|
|
Cement prod./gas flaring
|
68
|
172
|
192
|
65
|
104
|
141
|
206
|
249
|
136
|
479
|
36
|
187
|
64
|
224
|
462
|
Land- use change
|
1010
|
-139
|
-104
|
-902
|
-436
|
-139
|
-139
|
-139
|
2
|
81
|
-646
|
-501
|
2
|
2
|
2
|
|
TOTAL |
7312
|
16789
|
15044
|
8367
|
10983
|
12601
|
21802
|
21086
|
14397
|
28493
|
4147
|
13634
|
4789
|
30909
|
32988
|
|
Demand Side |
|
|
|
|
|
|
|
|
|
|
|
|
|
|
|
Residential/commercial
|
1995
|
7212
|
4631
|
3126
|
4059
|
4846
|
10290
|
7032
|
9407
|
7827
|
1698
|
5830
|
1209
|
14971
|
10939
|
Industry **
|
2784
|
3916
|
5734
|
1975
|
4042
|
2996
|
4388
|
4434
|
1471
|
9165
|
601
|
4909
|
1244
|
4341
|
3493
|
Transportation
|
1523
|
5800
|
4783
|
4168
|
3318
|
4897
|
7262
|
9759
|
3516
|
11421
|
2494
|
3396
|
2334
|
11596
|
18554
|
Land-use change
|
1010
|
-139
|
-104
|
-902
|
-436
|
-139
|
-139
|
-139
|
2
|
81
|
-646
|
-501
|
2
|
2
|
2
|
|
TOTAL |
7312
|
16789
|
15044
|
8367
|
10983
|
12601
|
21802
|
21086
|
14397
|
27493
|
4147
|
13634
|
4789
|
30909
|
32988
|
|
By Source |
|
|
|
|
|
|
|
|
|
|
|
|
|
|
|
Solids
|
2346
|
5356
|
7106
|
841
|
2230
|
2643
|
7553
|
12471
|
6166
|
22586
|
565
|
7765
|
188
|
3904
|
29596
|
Liquids
|
2787
|
5618
|
4100
|
3832
|
4542
|
5007
|
7310
|
4185
|
2751
|
933
|
910
|
1039
|
1542
|
7813
|
1118
|
Gases
|
1102
|
5782
|
3750
|
4532
|
4544
|
4949
|
6872
|
4320
|
5342
|
4415
|
3283
|
5144
|
2993
|
18967
|
1810
|
Others***
|
1078
|
33
|
88
|
-837
|
-332
|
3
|
67
|
110
|
138
|
559
|
-611
|
314
|
66
|
226
|
464
|
|
TOTAL |
7312
|
16789
|
15044
|
8367
|
10983
|
12601
|
21802
|
21086
|
14397
|
28493
|
4147
|
43634
|
4789
|
30909
|
32988
|
|
* Includes emissions from district- heat production,
energy transmission/ distribution, oil refining, fuel extraction,
and other conversion losses.
** Includes emissions from feedstocks, cement production, and gas
flaring.
*** Emissions from land- use change, cement production, and gas flaring. |
|
|