4.3.2 Opportunities in Forests
Many silvicultural and forest management practices have been reported to enhance
carbon mitigation (Lunnan et al., 1991; Hoen and Solberg, 1994; Karjalainen,
1996; Row, 1996; Binkley et al., 1997; Price et al. 1998; Birdsey et al., 2000;
Fearnside, 1999; Anonymous, 1999; Nabuurs et al., 2000). Measures suggested
for forests include: protecting against fires; protecting from disease, pests,
insects, and other herbivores; changing rotations; controlling stand density;
enhancing available nutrients; controlling the water table; selecting useful
species and genotypes; using biotechnology; reducing regeneration delays; selecting
appropriate harvest methods such as reduced-impact logging; managing logging
residues; recycling wood products; increasing the efficiency with which forest
products are manufactured and used; and establishing, maintaining, and managing
reserves.
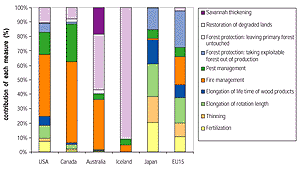
Figure 4.8: Relative importance of each of the 10 forest management
alternatives in the total potential sequestration as given in Figure
4.7. These data give an indication of opportunities and do not necessarily
represent national plans. For example, silvicultural practices in Japan
generally do not accompany fertilization and the figure for Japan is probably
an overestimate. Nevertheless it shows that opportunities vary among countries
because of both the national situation, the mix of current forestry practices,
and/or the historic management. One common recommendation of which measures
would yield the largest carbon sequestration can therefore not be given
(adapted from Nabuurs et al., 2000).
|
Sampson et al. (2000) provide an overview of the potential
impacts of some different management alternatives on carbon mitigation, and
examine both additional benefits and some possible unintended, negative effects
of these practices. They estimate that 10% of the global forest area could be
technically available by the year 2010, and that the global potential of forest
management practices could be 0.17GtC/yr. These opportunities rise to 50% of
the global forest area and 0.7GtC/yr by the year 2040. Sampson et al. (2000)
emphasize win-win situations, but also indicate the low level of certainty associated
with their estimates and the possibility for certain negative impacts.
Nabuurs et al. (2000) also estimate the potential of a broad range of forest-related
activities (including protection from natural disturbance, improved silviculture,
savannah thickening, restoration of degraded lands, and management of forest
products) at 0.6GtC/yr over six regions in the temperate and boreal zone (Canada,
USA, Australia, Iceland, Japan, and EU, Figure 4.7).
According to their estimates, alternative forest management for C sequestration
is technically feasible on 10% (on average) of the forest area in each region
examined. Figure 4.8 shows that the relative importance
of the different practices for the various regions depends on the current situation
in the respective regions.
The analyses of Sampson et al. (2000) and Nabuurs et al. (2000) estimate that
the hectare-scale effectiveness of these activities ranges from 0.02tC/ha/yr for
forest fertilization to 1.2tC/ha/yr for several practices combined in Loblolly
pine stands. However, they show that the impact of most practices is in the range
of 0.30.7tC/ha/yr.
Forest management and protection offer high mitigation potential in some countries.
For example, additional pools of 40-160tC/ha and 215tC/ha may be possible in
Cameroon and the Philippines, respectively (Sathaye and Ravindranath, 1998).
Afforestation or plantation forest options have the potential to increase carbon
stocks by 70100tC/ha in many places, and the potentials for some commercial
plantations may be even higher: 165tC/ha for timber estates in Indonesia, 120tC/ha
for timber forestry in India, and 236tC/ha for long rotation forestry in the
Philippines (Sathaye and Ravindranath, 1998). The suggested opportunities for
mitigation potential in 12 developing countries are summarized in Table
4.3.
The study of Sathaye and Ravindranath (1998) suggests that, in 10 tropical
and temperate countries in Asia, about 300Mha may be available for mitigation
options: 40Mha for conservation, protection, and management; 79Mha of degraded
forest land for regeneration; and 181Mha of degraded land for plantation forestry
and, hence, for C sequestration (Sathaye and Ravindranath, 1998). A further
172Mha was estimated to be available in these countries for agroforestry. These
estimates are much larger than those in IPCC (1996) (Table
4.4).
Table 4.4: Land categories and extent of
availability for mitigation in selected developing countries
(Sathaye and Ravindranath, 1998) |
 |
Country |
Forest land for
|
Degraded
|
Degraded land
|
Agroforestry
|
Others
|
Total
|
Area under
|
|
conservation,
|
forest land
|
for plantation
|
|
|
geographic
|
forests
|
|
protection, and
|
for regeneration
|
forestry
|
|
|
area
|
|
|
management
|
|
|
|
|
|
|
|
(Mha)
|
(Mha)
|
(Mha)
|
(Mha)
|
(Mha)
|
(Mha)
|
(Mha)
|
 |
|
|
|
|
|
|
|
|
Asia |
|
|
|
|
|
|
|
China |
|
19.2
|
105.2
|
75.9
|
|
932.6
|
134.0
|
India |
|
36.9
|
41.3
|
96.0
|
|
329.0
|
.63.3
|
Indonesia |
|
|
30.5
|
|
|
193.0
|
144.7
|
Mongolia |
|
2.4
|
1.6
|
|
|
156.6
|
17.5
|
Myanmar |
3.3
|
6.9
|
|
|
|
65.8
|
49.3
|
Pakistan |
0.5
|
0.3
|
2.6
|
1.2
|
|
77.1
|
3.7
|
Philippines |
6.6
|
2.5
|
|
|
0.60
|
29.8
|
6.5
|
South Korea |
0.7
|
0.3
|
|
|
0.05
|
9.9
|
6.5
|
Thailand |
17.8
|
4.4
|
|
|
|
51.1
|
14.0
|
Vietnam |
10.5
|
6.0
|
|
|
2.50
|
32.5
|
19.0
|
 |
Total |
39.4
|
78.9
|
181.2
|
171.9
|
3.15
|
1877.4
|
458.5
|
 |
|
|
|
|
|
|
|
|
Africa |
|
|
|
|
|
|
|
Cameroon |
1.6
|
|
7.3
|
1.6
|
|
46.0
|
36.0
|
Ghana |
0.9
|
|
0.3
|
2.5
|
|
23.0
|
18.0
|
|
Total |
2.5
|
0
|
7.6
|
4.1
|
0
|
69.0
|
54.0
|
|
Total (12 countries) |
41.9
|
78.9
|
188.8
|
176
|
3.15
|
1946.4
|
512.5
|
 |
Current estimates suggest that the cumulative C mitigation potential of forests
in 10 Asian countries is about 26.5GtC, suggesting that the SAR estimates for
the tropical region were conservative. China (9.7GtC) and India (8.7GtC) have
particularly large mitigation potentials in the forestry sector (Sathaye and
Ravindranath, 1998).
Latin America, which accounts for 51% of the global area of tropical forests
(FAO, 1997), has an estimated mitigation potential of at least 9.7GtC, an estimate
based on analyses of Mexico, Venezuela, and partly Brazil (Table
4.5). This total includes native forest management, protected areas, commercial
plantations, agroforestry, and restoration plantations. The technical potential
C mitigation in forestry is estimated at about 4.8GtC for Mexico, 1.4GtC for
Venezuela, and 3.5GtC for Brazil (Da Motta et al., 1999, Table
4.5). The feasible mitigation potential, which is largely constrained by
land tenure policies and socio-economic pressures (land availability), is, however,
often much lower than this technical potential. The feasible socio-economic
mitigation potential is about 50% less than the technical potential in Mexico
and about 44% lower than the technical potential in India (Ravindranath and
Somashekar, 1995).
Deforestation in the Brazilian Amazon is a significant source of CO2
and, with 90% of the originally forested area still uncleared, Brazil remains
a large potential source of future emissions. The deforestation rate in Amazonia
was estimated to be 1.38 million ha/yr in 1990, corresponding to an emission
of 251MtC/yr (Fearnside, 1997). The rate of deforestation has increased in recent
years, to 2.91Mha/yr in 1995 and 1.82Mha/yr in 1996 (Fearnside, 1998). Reducing
the deforestation rate by 50% would conserve 125MtC/yr. Thus, Brazil alone offers
a large potential for mitigation through slowing of deforestation.
What is the permanence of C sequestered by forest management activities? Clearly,
tree plantations that are harvested and not re-established do not contribute
to long-term carbon sequestration, though they may reduce atmospheric C in the
short term. But, if a new forest is maintained so that harvest equals net growth,
the forest can both be a source of wood products and still retain the captured
C. In other words, the sequestration phase may be finite, lasting only a few
decades, but the conservation phase need not be finite. Although there is an
exchange of carbon between the atmosphere and the biomass, a considerable pool
of carbon can be permanently stored in the steady-state biomass while wood products
continue to be produced. This C pool remains withdrawn from the atmosphere as
long as the forest exists. The substitution phase, which begins at the onset
of the first harvest, can be sustained. Each timber crop, in a cumulative manner,
can substitute for fossil-fuel resources. The forest thus offers a sustainable
alternative to the unsustainable use of fossil-fuel resources (Schlamadinger
and Marland, 1996).
Land owners are unlikely to manage their forest resources for C sequestration
alone. In the absence of financial incentives, any C sequestration will likely
be incidental, or have the role of a by-product in the management of forests
to produce valued goods and services (ITTA, 1983, 1994). In the tropical biome,
the optimal mix of management strategies will likely reflect a balance between
various forest management systems and agricultural production. Existing policies
for forest and agricultural land management, however, do not yet reflect economic
incentives for C management and probably are not optimal (see for example Poore
et al., 1989).
The effectiveness of various strategies for C sequestration will depend on
the initial status of the forest ecosystems. For lands without tree cover, afforestation
permits large C gains per hectare (Dyson, 1977; Sedjo and Solomon, 1989). Industrial
plantation forests are already being created on a large scale and expansion
of this area for C sequestration is possible (Sedjo and Sohngen, 2000). The
establishment of forest plantations is generally the most reliable silvicultural
method for afforestation, reforestation, and sustainable regeneration (regeneration
soon after cutting). Plantation establishment can enhance productivity if desired
species are planted on suitable sites. Plantations can reduce the pressures
to degrade natural forests (Sedjo and Botkin, 1997). However, following the
harvest of a mature or old-growth forest, the land can remain a source of carbon
for many decades, even when it is regenerated (Hoen and Solberg, 1994; Cohen
et al., 1996; Schlamadinger and Marland, 1996; Bhatti et al., 2001). Therefore,
for primary and mature forests, conserving and protecting the existing C pools
is often the only mitigation option that yields near-term benefits.
Because of the diversity in the current global forest status and socio-economic
situation, the optimal mix of mitigation strategies will vary with country and
region, in both the tropics and the non-tropics. For many countries, slowing
or halting deforestation is a major opportunity for mitigation (e.g., Brazil:
Fearnside, 1998, and Mexico: Masera, 1995). In countries such as India, where
deforestation rates have declined to marginal levels, afforestation and reforestation
in the degraded forest and non-forest lands offer large mitigation opportunities
(Ravindranath and Hall, 1995). Ravindranath and Hall (1995) have shown the potential
of using this degraded land and small biomass gasifiers to sustainably produce
electricity from woody biomass and displace 40 million tonnes of C annually.
In Africa an important opportunity for mitigation is in conserving wood fuel
and charcoal through improved efficiencies of stoves and charcoal kilns (Makundi,
1998). The selection of mitigation strategies or projects in tropical countries,
particularly, will be determined by economic development priorities, changing
pressures on land use, and resource constraints. In many industrialized countries,
adjusting forest management regimes and material flows in the forest products
sector (including substitution) appears most promising (Hoen and Solberg, 1994;
Binkley et al., 1997).
To quantify accurately the effects of changes in forest management on the net
transfer of C to the atmosphere, the whole system could be considered (see Box.
4.1). Many earlier studies focused on the immediate results of forest management
measures, e.g. the higher biomass growth rate following a silvicultural treatment
or the protected stock of C if wildfire or logging is prevented. Global assessments
based on these studies (e.g., Dixon et al., 1994; Brown et al., 1996b) have
limitations. Estimates, in terms of tC/ha or tC/ha/yr, leave unanswered the
critical questions of the timing, security, and sustainability of these effects.
Also, recent, more comprehensive studies indicate the importance of complete
accounting for all the C flows in and out of the system and the analysis of
long-term patterns. For example, Schlamadinger and Marland (1996) showed that
the positive effect of short-rotation plantations for fossil fuel substitution
is less than implied by the simple substitution of fossil fuels, because of
the continued input of fossil fuels needed to operate the system. While the
limitations of earlier studies are now evident, data for comprehensive analysis
at the global scale are not yet available. This, in part, explains why global-level
estimates of the potential for C mitigation in forestry remain unchanged from
those in SAR.
|