3.3 Sectoral Mitigation Technological Options7
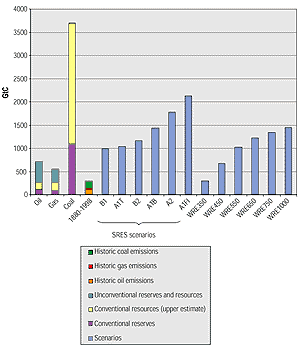
Figure TS.5: Carbon in oil, gas and coal reserves and resources
compared with historic fossil fuel carbon emissions 1860-1998, and with
cumulative carbon emissions from a range of SRES scenarios and TAR stabilization
scenarios up until 2100. Data for reserves and resources are shown in
the left hand columns. Unconventional oil and gas includes tar sands,
shale oil, other heavy oil, coal bed methane, deep geopressured gas, gas
in acquifers, etc. Gas hydrates (clathrates) that amount to an estimated
12,000 GtC are not shown. The scenario columns show both SRES reference
scenarios as well as scenarios which lead to stabilization of CO2
concentrations at a range of levels. Note that if by 2100 cumulative emissions
associated with SRES scenarios are equal to or smaller than those for
stabilization scenarios, this does not imply that these scenarios equally
lead to stabilization.
|
The potential8
for major GHG emission reductions is estimated for each sector for a range of
costs (Table TS.1). In the industrial sector, costs for
carbon emission abatement are estimated to range from negative (i.e.,
no regrets, where reductions can be made at a profit), to around US$300/tC9.
In the buildings sector, aggressive implementation of energy-efficient technologies
and measures can lead to a reduction in CO2 emissions from residential
buildings in 2010 by 325MtC/yr in developed and EIT countries at costs ranging
from -US$250 to US$150/tC and by 125MtC in developing countries at costs
of US$250 to US$50/tC. Similarly, CO2 emissions from commercial
buildings in 2010 can be reduced by 185MtC in developed and EIT countries at
costs ranging from US$400 to US$250/tC avoided and by 80MtC in developing
countries at costs ranging from -US$400 to US$0/tC. In the transport sector
costs range from US$200/tC to US$300/tC, and in the agricultural sector
from US$100/tC to US$300/tC. Materials management, including recycling
and landfill gas recovery, can also produce savings at negative to modest costs
under US$100/tC. In the energy supply sector a number of fuel switching and
technological substitutions are possible at costs from US$100 to more
than US$200/tC. The realization of this potential will be determined by the
market conditions as influenced by human and societal preferences and government
interventions.
Table TS.2 provides an overview and links with barriers
and mitigation impacts. Sectoral mitigation options are discussed in more detail
below.
3.3.1 The Main Mitigation Options in the Buildings Sector
The buildings sector contributed 31% of global energy-related CO2
emissions in 1995, and these emissions have grown at an annual rate of 1.8%
since 1971. Building technology has continued on an evolutionary trajectory
with incremental gains during the past five years in the energy efficiency of
windows, lighting, appliances, insulation, space heating, refrigeration, and
air conditioning. There has also been continued development of building controls,
passive solar design, integrated building design, and the application of photovoltaic
systems in buildings. Fluorocarbon emissions from refrigeration and air conditioning
applications have declined as chlorofluorocarbons (CFCs) have been phased out,
primarily thanks to improved containment and recovery of the fluorocarbon refrigerant
and, to a lesser extent, owing to the use of hydrocarbons and other non-fluorocarbon
refrigerants. Fluorocarbon use and emission from insulating foams have declined
as CFCs have been phased out, and are projected to decline further as HCFCs
are phased out. R&D effort has led to increased efficiency of refrigerators
and cooling and heating systems. In spite of the continued improvement in technology
and the adoption of improved technology in many countries, energy use in buildings
has grown more rapidly than total energy demand from 1971 through 1995, with
commercial building energy registering the greatest annual percentage growth
(3.0% compared to 2.2% in residential buildings). This is largely a result of
the increased amenity that consumers demand in terms of increased use
of appliances, larger dwellings, and the modernization and expansion of the
commercial sector as economies grow. There presently exist significant
cost-effective technological opportunities to slow this trend. The overall technical
potential for reducing energy-related CO2 emissions in the buildings
sector using existing technologies combined with future technical advances is
715MtC/yr in 2010 for a base case with carbon emissions of 2,600MtC/yr (27%),
950MtC/yr in 2020 for a base case with carbon emissions of 3,000MtC/yr (31%),
and 2,025MtC/yr in 2050 for a base case with carbon emissions of 3,900MtC/yr
(52%). Expanded R&D can assure continued technology improvement in this
sector.
Table TS. 1: Estimations of greenhouse gas
emission reductions and cost per tonne of carbon equivalent avoided following
the anticipated socio- economic potential uptake by 2010 and 2020 of selected
energy efficiency and supply technologies, either globally or by region
and with varying degrees of uncertainty |
 |
|
|
US$/ tC avoided
|
2010
|
2020
|
References, comments, and relevant
section in Chapter 3 of this report |
|
Region |
400
|
200
|
0
|
+200
|
Potentiala
|
Probabilityb
|
Potentiala
|
Probabilityb
|
 |
Buildings/appliances |
|
|
|
|
|
|
|
Residential sector |
OECD/ EIT |
 |
 |
 |
 |
 |
Acosta Moreno et al., 1996;
Brown et al., 1998 |
Dev. cos.31 |
 |
 |
 |
 |
 |
Wang and Smith, 1999 |
Commercial sector |
OECD/ EIT |
 |
 |
 |
 |
 |
|
Dev. cos. |
 |
 |
 |
 |
 |
|
|
|
|
|
|
|
|
|
Transport |
|
|
|
|
|
|
Automobile efficiency improvements |
USA |
 |
 |
 |
 |
 |
Interlab. Working Group, 1997
Brown et al., 1998 |
Europe |
 |
 |
 |
 |
 |
US DOE/ EIA, 1998
ECMT, 1997 (8 countries only) |
Japan |
 |
 |
 |
 |
 |
Kashiwagi et al., 1999
Denis and Koopman, 1998 |
|
Dev. cos. |
 |
 |
 |
 |
 |
Worrell et al., 1997b |
|
|
|
|
|
|
|
|
Manufacturing |
|
|
|
|
|
|
|
CO2 removal fertilizer; refineries |
Global |
 |
 |
 |
 |
 |
Table 3.21 |
Material efficiency improvement |
Global |
 |
 |
 |
 |
 |
Table 3.21 |
Blended cements |
Global |
 |
 |
 |
 |
 |
Table 3.21 |
N2O reduction by chem. indus. |
Global |
 |
 |
 |
 |
 |
Table 3.21 |
PFC reduction by Al industry |
Global |
 |
 |
 |
 |
 |
Table 3.21 |
HFC-23 reduction by chem. industry |
Global |
 |
 |
 |
 |
 |
Table 3.21 |
Energy efficient improvements |
Global |
 |
 |
 |
 |
 |
Table 3.19 |
|
|
|
|
|
|
|
|
Agriculture |
|
|
|
|
|
|
Increased uptake of conservation tillage and cropland
management |
Dev. cos. |
 |
 |
 |
 |
 |
Zhou, 1998; Table 3.27
Dick et al ., 1998 |
Global |
 |
 |
 |
 |
 |
IPCC, 2000 |
Soil carbon sequestration |
Global |
 |
 |
 |
 |
 |
Lal and Bruce, 1999
Table 3.27 |
Nitrogenous fertilizer management |
OECD |
 |
 |
 |
 |
 |
Kroeze & Mosier, 1999
Table 3.27 |
Global |
 |
 |
 |
 |
 |
OECD, 1999; IPCC, 2000 |
Enteric methane reduction |
OECD |
 |
 |
 |
 |
 |
Kroeze & Mosier, 1999
Table 3.27 |
USA |
 |
 |
 |
 |
 |
OECD, 1998
Reimer & Freund, 1999 |
Dev. cos. |
 |
 |
 |
 |
 |
Chipato, 1999 |
Rice paddy irrigation and fertilizers |
Global |
 |
 |
 |
 |
 |
Riemer & Freund, 1999
IPCC, 2000 |
|
|
|
|
|
|
|
|
Wastes |
|
|
|
|
|
|
|
Landfill methane capture |
OECD |
 |
 |
 |
 |
 |
Landfill methane USEPA, 1999 |
|
|
|
|
|
|
|
|
Energy supply |
|
|
|
|
|
|
|
Nuclear for coal |
Global |
|
 |
 |
 |
 |
Totalsc See Section
3.8.6 |
Annex I |
 |
 |
 |
 |
 |
Table 3.35a |
Non- Annex I |
 |
 |
 |
 |
 |
Table 3.35b |
Nuclear for gas |
Annex I |
 |
 |
 |
 |
 |
Table 3.35c |
Non- Annex I |
 |
 |
 |
 |
 |
Table 3.35d |
Gas for coal |
Annex I |
 |
 |
 |
 |
 |
Table 3.35a |
Non- Annex I |
 |
 |
 |
 |
 |
Tables 3.35b |
CO2 capture from coal |
Global |
 |
 |
 |
 |
 |
Tables 3.35a + b |
CO2 capture from gas |
Global |
 |
 |
 |
 |
 |
Tables 3.35c + d |
Biomass for coal |
Global |
 |
 |
 |
 |
 |
Tables 3.35a + b
Moore, 1998; Interlab w. gp. 1997 |
Biomass for gas |
Global |
 |
 |
 |
 |
 |
Tables 3.35c + d |
Wind for coal or gas |
Global |
 |
 |
 |
 |
 |
Tables 3.35a - d
BTM Cons 1999; Greenpeace, 1999 |
Co-fire coal with 10% biomass |
USA |
 |
 |
 |
 |
 |
Sulilatu, 1998 |
Solar for coal |
Annex I |
 |
 |
 |
 |
 |
Table 3.35a |
Non- Annex I |
 |
 |
 |
 |
 |
Table 3.35b |
Hydro for coal |
Global |
 |
 |
 |
 |
 |
Tables 3.35a + b |
Hydro for gas |
Global |
 |
 |
 |
 |
 |
Tables 3.35c + d |
 |
|
|