Executive Summary
The technological and economic potential to reduce greenhouse gas (GHG) emissions
is large enough to hold annual global greenhouse gas emissions to levels close
to or even below those of 2000 by 2010 and even lower by 2020. Realization of
these reductions requires combined actions in all sectors of the economy including
adoption of energy-efficient technologies and practices, increased fuel switching
toward lower carbon fuels, continued growth in the use of efficient gas turbines
and combined heat and power systems, greater reliance on renewable energy sources,
reduced methane emissions through improved farm management practices and ruminant
methane reduction strategies, diversification of land use to provide sinks and
offsets, increased recovery of landfill methane for electricity production and
increased recycling, reduction in the release of industrial gases, more efficient
vehicles, physical sequestration of CO2, and improving end-use efficiency
while protecting the ozone layer. Countervailing socioeconomic and behavioural
trends that cause greenhouse gas emissions to increase also exist, including
increased size of dwelling units, increased sales of heavier and more powerful
vehicles, growing vehicle kilometers travelled, reduced incentives for efficient
use of energy or the purchase of energy efficiency technologies as a result
of low real retail energy prices, increased consumption of consumer goods, and
stimulated demand for energy-consuming products as a result of increased electrification.
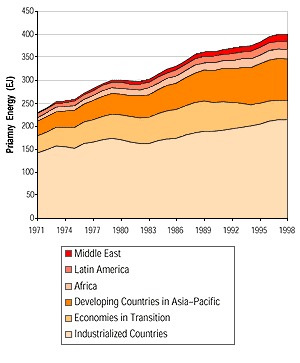
Figure 3.1: World primary energy use per region 1971 - 1998.
Note: Primary energy calculated using the IEA�s physical energy content
method based on the primary energy sources used to produce heat and electricity
(IEA, 2000).
|
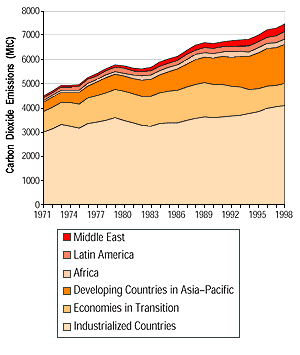
Figure 3.2: World CO2 emissions by region, 1971 - 1998.
|
A number of new technologies and practices have gained importance since the
Second Assessment Report (SAR). As a result, greater opportunities for energy
efficiency are available, often at lower cost than was expected. Annual growth
in global consumption of primary energy and related carbon dioxide emissions
dropped to 1.3% and 1.4%, respectively, between 1990 and 1998 after experiencing
much higher growth rates of 2.4% and 2.1% between 1971 and 1990. This decrease
in growth rate is because of the combined effects of improved energy efficiency
technologies, increased fuel switching and adoption of renewable energy sources,
and the dramatic decrease in emissions of countries with economies in transition
(EITs) as a result of economic changes (Figures 3.1 and
3.2).
Sustained progress in the development and adoption of technologies and practices
to reduce greenhouse gas emissions requires continued efforts in the areas of
research and development, demonstration, dissemination, policies, and programmes.
There has been a reduction in both public and private resources devoted to research
and development to develop and implement new technologies that will reduce greenhouse
gas emissions. Despite the development of new, efficient technologies, current
rates of energy efficiency improvements alone will not be sufficient to significantly
reduce greenhouse gas emissions in the near term. In addition, policies or programmes
to increase energy efficiency and promote renewable energy technology are lacking
in many countries.
Technological innovation and change are influenced by the differing needs of
different economies and sectors. A large percentage of capital is invested in
a relatively small number of technologies that are responsible for a significant
share of the energy supply and consumption market (automobiles, electric power
generators, industrial processes, and building heating and cooling systems).
There is a tendency to optimize these few technologies and their related infrastructure
development, gaining them advantages and locking them into the economy. That
makes it more difficult for alternative, low-carbon technologies to compete.
For example, a particular technological configuration such as road-based automobiles
has become locked-in as the dominant transportation mode. In industrial
countries, technologies are developed as a result of corporate innovation or
government-supported R&D, and in response to environmental regulations,
utility deregulation, energy tax policies, or other incentives. In many developing
countries, where electric power capacity and much end-use demand is growing
most rapidly, there is often greater emphasis on getting technology such as
electric power generation established in order to enhance economic development,
with less concern for environmental and other issues. Capital flows and differing
types of technology transfer may also determine technology choices. It is important
to recognize that often values other than energy efficiency or greenhouse gas
emissions are the dominant shapers of technological choice and innovation.
This chapter describes technologies and practices to reduce greenhouse gas
emissions in the end-use sectors of the economy as well as through changes in
energy supply. The end-use sectors addressed are buildings, transport, industry,
agriculture, and waste. Energy supply includes non-renewable resources, renewable
resources, and physical carbon dioxide sequestration. In addition, options for
reducing global warming contributions from substitutes for ozone depleting substances
are discussed in the Appendix to this chapter.
The buildings sector contributes about 31% of global energy-related carbon
dioxide emissions and these emissions grew at an average annual rate of almost
2.0% between 1971 and 1995. Growth in emissions varied significantly by region;
between 1990 and 1995 the largest annual increases were experienced in developing
countries (around 5.0% per year), moderate growth was seen in developed countries
(around 1.0% per year), and emissions declined in the EITs (3.0% per year).
The growth in emissions in the developing and developed countries is largely
caused by the increased amenity that consumers demand in terms of increased
purchase and use of appliances, larger dwellings, and the modernization and
expansion of the commercial sector as economies grow. Technology has
continued on an evolutionary trajectory with incremental gains during the past
decade in windows, lighting, insulation, space heating, refrigeration, air conditioning,
building controls, passive solar design, and infiltration reduction. Although
CFCs have been eliminated in developed countries as working fluids in heat pumps,
air conditioners, and refrigerators, and as foam blowing agents for insulation,
research and development (R&D) has been able to continue to improve energy
efficiency of refrigerators and cooling and heating systems. Integrated building
design has demonstrated very large reductions in energy use and greenhouse gas
emissions. Expanded R&D is needed to assure continued technology improvement,
but implementation policies remain the major hurdle to their rapid introduction.
The transport sector contributes 22% of carbon dioxide emissions; globally,
emissions from this sector are growing at a rate of approximately 2.5% annually.
Between 1990 and 1995, growth was highest in the developing countries (7.3%
per year in the developing countries of AsiaPacific and 4.6% per year
in the remaining developing countries), moderate in the developed countries
(1.9% per year) and is actually declining at a rate of 5.0% per year for
the EITs. Technology improvements may generate operational cost reductions that
have a rebound effect that stimulates further personal transportation use. These
issues show the necessity of both policies and behavioural changes to lower
emissions from the transport sector. Hybrid gasoline-electric vehicles have
been introduced on a commercial basis with fuel economies 50% to 100% better
than that of comparably sized four-passenger vehicles. The development of extremely
low-polluting engines may reduce the incentive for hybrid and battery electric
vehicles that were previously thought to encourage the adoption of vehicles
that would also reduce greenhouse gases. Lightweight materials have the potential
to improve fuel economy for all land transport. Fuel cell powered vehicles are
developing rapidly, and could be introduced to the market sometime during the
coming decade. Substantial potential for improving the fuel economy of heavy-duty
trucks seems feasible. Only incremental improvements of the order of 1%/yr are
expected for aircraft over the next several decades. There appears to be little
attention being given to rail or public transportation systems, but waterborne
transport of freight is already highly efficient, and has potential for additional
gains.
Industrial emissions account for over 40% of carbon dioxide emissions. Global
industrial sector carbon dioxide emissions grew at a rate of 1.5% per year between
1971 and 1995, slowing to 0.4% per year between 1990 and 1995. This is the only
sector that has shown an annual decrease in carbon emissions in industrial economies
(0.8% per year between 1990 and 1995) as well as in the EITs (6.4%
per year between 1990 and 1995). Emissions from this sector in developing countries,
however, continue to grow (6.3% per year in developing countries of AsiaPacific
and 3.4% per year in the remaining developing countries). Substantial differences
in the energy efficiency of industrial processes between countries exist. Improvement
of energy efficiency is the most important emission reduction option in the
short term. However, industries continue to find new, more energy efficient
processes which makes this option also important for the longer term. The larger
part of the energy can be saved at net negative costs. In addition, material
efficiency improvement (including more efficient product design, recycling,
and material substitution) can greatly contribute to reducing emissions. For
many sources of non-CO2 emissions, like those from the aluminium
industry, and adipic acid and HCFC-22 production, substantial emission reductions
are possible or are already being implemented.
The agricultural sector has the smallest direct CO2 emissions, contributing
4.0% of total global emissions. Growth in these emissions between 1990 and 1995
was greatest in the developing countries (6.0% per year in the developing countries
of AsiaPacific and 9.3% per year in the remaining developing countries),
modest in the developed countries (1.3% per year), and declined at a rate of
5.4% per year in the EIT. However, methane and nitrous oxide emissions
dominate the agricultural sector, which contributes over 20% of global anthropogenic
greenhouse gas emissions in terms of CO2 equivalents. Reductions
can be made by improved farm management practices such as more efficient fertilizer
use, better waste treatment, use of minimum tillage techniques, and ruminant
methane reduction strategies. Biotechnology and genetic modification developments
could provide additional future gains and also lead to reduced energy demand,
but the conflict between food security and environmental risk is yet to be resolved.
Mitigation solutions exist overall for 100200MtCeq/yr but farmers
are unlikely to change their traditional farming methods without additional
incentives. Diversification of land use to energy cropping has the technical
potential to provide both carbon sinks and offsets in regions where suitable
land and water are available. Transport biofuel production costs remain high
compared with oil products, but do provide additional value in the form of oxygenates
and increased octane (ethanol). Because of market liberalization policies, the
potential for biofuels has declined, though there is a growing demand for biodiesel
in Germany. Improvements in biofuel conversion routes, such as the enzymatic
hydrolysis of lignocellulosic material to ethanol, may help narrow the cost
disadvantage versus fossil fuels.
Greenhouse gas emissions are being lowered substantially by increased utilization
of methane from landfills and from coal beds for electric power generation.
Significant energy-related greenhouse gas reductions are identified for improved
waste recycling in the plastics and carpet industries, and through product remanufacturing.
A major discussion is taking place over whether the greater reduction in lifecycle
CO2 emissions occurs through paper recycling or by utilizing waste
paper as a biofuel in waste to energy facilities. In several developed countries,
and especially in Europe and Japan, waste-to-energy facilities have become more
efficient with lower air pollution emissions.
Abundant fossil fuel reserves that are roughly five times the total carbon
already burned are available. The electric power sector accounts for 38% of
total CO2 emissions. Low cost, aero-derivative, combined cycle gas
turbines with conversion efficiencies approaching 60% have become the dominant
option for new electric power generation plants, wherever adequate natural gas
supply and infrastructure are available. With deregulation of the electric power
sector, additional emission reductions have occurred in most countries through
the utilization of waste heat in combined heat and power systems that are capable
of utilizing 90% of the fossil fuel energy. Low carbon- emitting technologies
such as nuclear power have managed to significantly increase their capacity
factor at existing facilities, but relatively few new plants are being proposed
or built because of public concern about safety, waste storage, and proliferation.
There has also been rapid deployment of wind turbines and smaller, but expanding
markets for photovoltaic solar power systems. The annual growth rate from a
small base for both wind and solar currently exceeds 25% per year, and together
with an increasing number of bioenergy plants, accounts for around 2% of global
electricity generation. Modern biomass gasification is increasing the opportunities
for this renewable resource. There remains additional hydropower potential in
some locations, but most large sites have already been developed in many regions
of the world. Fuel cells appear to be a promising combined heat and electric
power source as part of evolving distributed generation systems.
Further analysis since the SAR suggests that physical sequestration of CO2
underground in aquifers, in depleted gas and oil fields, or in the deep ocean
is potentially a viable option. Technical feasibility has been demonstrated
for CO2 removal and storage from a natural gas field, but long-term
storage and economic viability remain to be demonstrated. Environmental implications
of ocean sequestration are still being evaluated. The utilization of hydrogen
from fossil fuels, biomass, or solid waste followed by sequestration appears
particularly attractive. Along with biological sequestration, physical sequestration
might complement current efforts at improving energy efficiency, fuel switching,
and the further development and implementation of renewables, but it must compete
economically with them.
Hydrofluorocarbon (HFCs) and perfluorocarbon (PFCs) use is growing as CFCs
and, to a much lesser extent HCFCs, are eliminated. There is a variety of uses
for these substances as alternatives in refrigeration, mobile and stationary
air-conditioning, heat pumps, in medical and other aerosol delivery systems,
insulating plastic foams, and for fire suppression and solvents. The replacement
of ozone-depleting substances with HFCs and PFCs has been about one-tenth on
a mass basis, with the difference being attributed to improved containment,
recovery of fluids, and the use of alternative substances. The importance of
considering energy efficiency simultaneously with ozone layer protection is
discussed in the Appendix, especially in the context
of developing countries.
This chapter concludes with a quantification of the potential for reducing
greenhouse gas (GHG) emissions in the various end-use sectors of the economy
and through changes in energy supply. It is found that sufficient technological
potential exists to stabilize or lower global greenhouse gas emissions by 2010,
and to provide for further reductions by 2020. The quantification is based on
sector-specific analyses and, thus, caution should be taken when adding up the
various estimates resulting from interactions between different types of technologies.
These sector-based analyses can be used to provide further understanding of
the results of global mitigation scenarios, such as those presented in Chapter
2, which account for inter-sectoral interactions, but typically do not provide
estimates of sectoral level GHG emissions reduction potential or costs.
Some of the costs associated with sector specific options for reducing GHG
emissions may appear high (for example US$300/tCeq). However, we
estimate that there is technological potential for reductions of between 1,900
and 2,600MtCeq/yr in 2010 and 3,600 to 5,050MtCeq/yr in
2020. Half of these reductions are achievable at net negative costs (value of
energy saved is greater than capital, operating and maintenance costs), and
most of the remainder is available at a cost of less than US$100tCeq/yr.
The continued development and adoption of a wide range of greenhouse gas mitigation
technologies and practices will result not only in a large technical and economic
potential for reducing greenhouse gas emissions but will also provide continued
means for pursuing sustainable development goals.
|