6.1.3. Aviation Scenarios Adopted for Climate Assessment
A few, detailed, three-dimensional (3-D) emission inventories for three specific
years-1992, 2015, and 2050-are presented in Chapter 9
and are studied with 3-D atmospheric models: NASA-1992, NASA-2015, and the ICAO-developed
FESGa and FESGe scenarios for 2050. The FESG scenarios include three economic
options, a/c/e, corresponding to economic growth assumed in IS92a/c/e. Each
of the FESG scenarios has technology option 1 assuming typical, market-driven
advances in engine/airframe technology and technology option 2 with advanced
engine technology (i.e., a 25% reduction in NOx emission
index with a 3.5% increase in fuel use; see Chapter 9).
Between these fixed-year scenarios, linear interpolation is used to derive continuous
scenarios-Fa1, Fa2, Fc1, and Fe1 (see Table 6-3)-that extend from 1990 to 2050.
CO2 increases are derived from carbon-cycle models (see
notes to Tables 6-1 and 6-2).
Two scenarios based on EDF projections for the years 2015 and 2050 (Vedantham
and Oppenheimer, 1998) provide only global CO2 and NOx
emissions: the EDF-a-base (Eab) and EDF-d-high (Edh) cases. The Edh scenario
was not adopted for its relationship to any underlying population or economic
scenario, but because it is a smooth extrapolation of recent growth rates. Atmospheric
changes other than CO2 for Eab and Edh are scaled from
the Fa1 and Fe1 scenarios (see notes to Table 6-1).
The continuous scenarios are summarized in Table 6-3.
Table 6-1: Aviation fixed-year (1992, 2015, and 2050) scenarios
for emissions and radiative forcing.
|
Scenario
|
Fuel Burn (Mt yr-1)
|
NOx Emis. d (Mt yr-1)
|
CO2f
Conc. (ppmv)
|
CO2f
|
R
O3g ..
|
adiative
CH4g
|
Forcing
H2Oh
|
(W m-2)
Contrailsi
|
Sulfateh Aerosols |
BCh Aerosols |
Total
|
RFI
|
NASA-1992*a
Lowb
High
|
160.3
|
1.92
|
1.0
|
+.018
+.013
+.023
|
+.023
+.011
+0.46
|
-.014
-.005
-.042
|
+.0015
+.000
+.005
|
+.020
+.005
+.06
|
-.003
-.001
-.009
|
+.003
+.001
+.009
|
+.048
|
2.7
|
NASA-2015*a
|
324.0
|
4.34
|
2.5
|
+.038
|
+.040
|
-.027
|
+.003
|
+.060
|
-.006
|
+.006
|
+.114
|
3.0
|
FESGa(tech1)50
Low
High
FESGa(tech2)50
|
471.0
 
 
487.6
|
7.15
 
 
5.55
|
6.0
 
 
6.1
|
+.074
+.052
+.096
+.075
|
+.060
+.030
+.120
+.047
|
-.045
-.015
-.120
-.035
|
+.004
+.000
+.015
+.005
|
+.100
+.03
+.40
+.100
|
-.009
-.003
-.027
-.009
|
+.009
+.003
+.027
+.009
|
1.93
 
 
1.92
|
2.6
 
 
 
|
FESGc(tech1)50
FESGc(tech2)50
|
268.2
267.2
|
4.01
3.14
|
4.9
5.0
|
+.060
+.061
|
+.034
+.026
|
-.025
-.020
|
+.003
+.003
|
+.057
+.057
|
-.005
-.005
|
+.005
+.005
|
+.129
+.127
|
2.2
 
|
FESGe(tech1)50
FESGe(tech2)50
|
744.3
772.1
|
11.38
8.82
|
7.4
7.6
|
+.091
+.093
|
+.096
+.074
|
-.072
-.055
|
+.007
+.007
|
+.158
+.158
|
-.014
-.015
|
+.014
+.015
|
+.280
+.277
|
3.1
 
|
EDFa-base 2015
EDFa-base 2050
|
297.0d
1143.0
|
2.85
7.89
|
2.4
9.4
|
+.037
+.115
|
+.026
+.066
|
-.018
-.050
|
+.003
+.011
|
+.055
+.243
|
-.006
-.022
|
+.006
+.022
|
+.103
+.385
|
2.8
3.3
|
EDFd-high 2015
EDFd-high 2050
|
448.0d
1688.0
|
4.30
11.65
|
3.0
13.4
|
+.046
+.165
|
+.040
+.098
|
-.027
-.073
|
+.004
+.016
|
+.083
+.358
|
-.008
-.032
|
+.008
+.032
|
+.146
+.564
|
3.2
3.4
|
HSCT (500)
Low
High
|
70.0
 
 
|
0.35
 
 
|
|
|
-.010
-.040
+.010
|
|
+.050
+.017
+.150
|
|
|
|
|
|
HSCT (1000)
Low
High
|
140.0
 
 
|
0.70
 
 
|
|
|
-.010
-.040
+.010
|
|
+.100
+.033
+.300
|
|
|
|
|
|
Net HSCT 2050
+HSCT
-subsonic
FESGa(tech1)
+HSCT 2050
|
 
+140.0
-53.6
 
557.4
|
 
+0.70
-0.81
 
7.04
|
 
+0.8
-0.3
 
6.5
|
 
+.010
-.004
 
+.080
|
 
-.010
-.007
 
+.043
|
 
 
+.005
 
-.040
|
 
+.100
-.001
 
+.103
|
 
 
-.011
 
+.089
|
 
 
+.001
 
-.008
|
 
 
-.001
 
+.008
|
 
+.100
-.018
 
+.275
|
 
 
 
 
3.4
|
|
a The scenarios
in boldface were studied in atmospheric models with defined 3-D emission patterns;
the others were scaled to these scenarios. The NASA-1992* aviation scenario
has been scaled here by 1.15, and the NASA-2015* scenario by 1.05, to account
for inefficiencies in flight routing.
b Low/High give likely (67% probability) range.
c In FESG scenarios, tech 1 is standard, and tech 2 reduces EI(NOx)
by 25% with a few percent additional fuel use.
d Throughout the table and this report, NOx emissions (Mt yr-1)
and indices (EI) use the NO2 molecular weight.
e In the EDF 2015 scenarios, the fuel burns have been revised to 374
(a-base) and 592 (d-high) Mt yr-1, which would increase
the added CO2 by 2050 to 10.0 (a-base) and 14.7 (d-high)
ppmv.
f CO2 is largely cumulative and depends on the
assumed previous history of the emissions; CH4 perturbations
are decadal in buildup time; all other perturbations reach steady-state balance
with emissions in a few years. All except CO2 are assumed
here to be instantaneous. Thus, CO2 concentrations are
based on complete history of fuel burn-for example, scenario Fa1 = NASA-1992*
' NASA-2015* ' FESGa (tech1) 2050; and scenario Eab = NASA-1992* ' EDFa-base
2015 ' EDFa-base 2050, all with linear interpolation between 1992, 2015, and
2050 (see also Section 6.1.3).
g The O3 and CH4 RFs are
scaled to NOx emissions for non-bold scenarios.
h As for note g, stratospheric H2O, sulfate,
and BC aerosols scale with fuel burn.
i Contrails do not scale with fuel burn as the fleet and flight routes
evolve (see Chapter 3). The contrail RF here is from line-shaped contrail cirrus
only. Additional induced cirrus cover RF is positive, and may be of similar
magnitude, but no best estimate can be given yet.
Table 6-2: Emissions, atmospheric concentrations, radiative
forcing, and climate change (global mean surface temperature) projected
for the years 1990, 2000, 2015, 2025, and 2050 using IPCC's IS92a and the
aviation scenarios from Tables 6-1 and 6-3.
|
|
1990
|
2000
|
2015
|
2025
|
2050
|
Emissions |
IS92a CO2 Emissions (Gt C yr-1) |
Fossil Fuel |
6.0
|
7.2
|
9.2
|
10.7
|
13.2
|
Total |
7.5
|
8.5
|
10.7
|
12.2
|
14.5
|
Aviation CO2 Emissions (Gt C yr-1) |
Fa1 |
0.147
|
0.187
|
0.279
|
0.315
|
0.405
|
Fa2 |
0.147
|
0.187
|
0.279
|
0.319
|
0.419
|
Fc1 |
0.147
|
0.187
|
0.279
|
0.265
|
0.231
|
Fe1 |
0.147
|
0.187
|
0.279
|
0.382
|
0.640
|
Eab |
0.147
|
0.179
|
0.255
|
0.463
|
0.983
|
Edh |
0.147
|
0.224
|
0.385
|
0.690
|
1.452
|
Fa1H |
0.147
|
0.187
|
0.279
|
0.344
|
0.479
|
IS92a NOx Emissions (Mt NO2
yr-1) |
Energy |
82
|
98
|
122
|
137
|
174
|
Biomass Burn |
30
|
31
|
32
|
33
|
36
|
Aviation NOx Emissions (Mt NO2
yr-1) |
Fa1 |
2.0
|
2.8
|
4.3
|
5.1
|
7.2
|
Fa2 |
2.0
|
2.8
|
4.3
|
4.7
|
5.6
|
Fc1 |
2.0
|
2.8
|
4.3
|
4.2
|
4.0
|
Fe1 |
2.0
|
2.8
|
4.3
|
6.4
|
11.4
|
Eab |
2.0
|
2.2
|
2.9
|
4.3
|
7.9
|
Edh |
2.0
|
2.8
|
4.3
|
6.4
|
11.6
|
Atmospheric Concentrations |
IS92a Atmosphere |
CO2 (ppmv) |
354
|
372
|
405
|
432
|
509
|
CH4 (ppbv) |
1700
|
1810
|
2052
|
2242
|
2793
|
N2O (ppbv) |
310
|
319
|
333
|
344
|
371
|
Aviation Marginal CO2 (ppmv) |
Fa1 |
0.9
|
1.5
|
2.5
|
3.5
|
6.0
|
Fa2 |
0.9
|
1.5
|
2.5
|
3.5
|
6.1
|
Fc1 |
0.9
|
1.5
|
2.5
|
3.2
|
4.9
|
Fe1 |
0.9
|
1.5
|
2.5
|
3.9
|
7.4
|
Eab
|
0.9
|
1.5
|
2.4
|
4.4
|
9.4
|
Edh |
0.9
|
1.7
|
3.0
|
6.0
|
13.4
|
Fa1H |
0.9
|
1.5
|
2.5
|
3.5
|
6.5
|
Aviation Marginal CH4 (ppbv) |
Fa1 |
-31
|
-49
|
-75
|
-97
|
-152
|
Radiative Forcing |
Differential RF (W m-2/ppmv) |
dRF/dCO2 |
0.018
|
0.016
|
0.015
|
0.014
|
0.012
|
dRF/dCH4 |
0.38
|
0.37
|
0.35
|
0.33
|
0.29
|
IS92a RF (Wm-2) |
CO2 |
1.54
|
1.84
|
2.38
|
2.79
|
3.83
|
NO4 |
0.47
|
0.51
|
0.59
|
0.66
|
0.83
|
N2O |
0.14
|
0.17
|
0.22
|
0.26
|
0.36
|
All greenhouse gases |
2.64
|
3.08
|
3.81
|
4.34
|
5.76
|
Aerosols (direct/indirect) |
-1.26
|
-1.36
|
-1.55
|
-1.66
|
-1.94
|
Total |
1.38
|
1.72
|
2.26
|
2.68
|
3.82
|
Aviation Fa1 Components of RF (Wm-2) |
CO2 |
0.016
|
0.025
|
0.038
|
0.048
|
0.074
|
O3 |
0.024
|
0.029
|
0.040
|
0.046
|
0.060
|
CH4 |
-0.015
|
-0.018
|
-0.027
|
-0.032
|
-0.045
|
H2O |
0.002
|
0.002
|
0.003
|
0.003
|
0.004
|
Contrails |
0.021
|
0.034
|
0.060
|
0.071
|
0.100
|
Sulfate aerosol |
-0.003
|
-0.004
|
-0.006
|
-0.007
|
-0.009
|
Soot (BC) aerosol |
0.003
|
0.004
|
0.006
|
0.007
|
0.009
|
Indirect clouds |
n.a.
|
n.a.
|
n.a.
|
n.a.
|
n.a.
|
Total |
0.048
|
0.071
|
0.114
|
0.137
|
0.193
|
Aviation HSCT (net) Components of RF (Wm-2) |
CO2 |
|
|
|
0.001
|
0.006
|
O3 |
|
|
|
-0.007
|
-0.017
|
CH4 |
|
|
|
0.002
|
0.005
|
H2O |
|
|
|
0.040
|
0.099
|
Contrails |
|
|
|
-0.004
|
-0.011
|
Total |
|
|
|
0.031
|
0.082
|
Aviation Scenarios Total RF (Wm-2) |
Fa1 |
0.048
|
0.071
|
0.114
|
0.137
|
0.193
|
Fa2 |
0.048
|
0.071
|
0.114
|
0.136
|
0.192
|
Fc1 |
0.048
|
0.071
|
0.114
|
0.118
|
0.129
|
Fe1 |
0.048
|
0.071
|
0.114
|
0.161
|
0.280
|
Eab |
0.048
|
0.068
|
0.103
|
0.184
|
0.385
|
Edh |
0.048
|
0.083
|
0.146
|
0.265
|
0.564
|
Fa1H |
0.048
|
0.071
|
0.114
|
0.168
|
0.275
|
Climate Change |
Global Mean Surface Air Temperature Change (K) |
IS92a |
0.000
|
0.140
|
0.360
|
0.510
|
0.920
|
Fa1 |
0.000
|
0.004
|
0.015
|
0.024
|
0.052
|
Fc1 |
0.000
|
0.004
|
0.015
|
0.023
|
0.039
|
Fe1 |
0.000
|
0.004
|
0.015
|
0.026
|
0.070
|
Eab |
0.000
|
0.004
|
0.014
|
0.026
|
0.090
|
Edh |
0.000
|
0.005
|
0.019
|
0.038
|
0.133
|
Fa1H |
0.000
|
0.004
|
0.015
|
0.025
|
0.066
|
Notes: The 1990
values are based on IEA data for 1990 fuel use; these values are higher
than our estimate for 1992 (see Table 6-1).
The scenarios involve linear interpolation of emissions between 1990, 1992,
2015, and 2050 (see Tables 6-1 and 6-3).
The projected CH4 increases are based on the emissions
growth in IS92a that do not match the much smaller trends currently observed.
These calculations used the methodologies from the IPCC's Second Assessment
Report (1996) as contributed by Atul Jain, Michael Prather, Robert Sausen,
Ulrich Schumann, Tom Wigley, and Don Wuebbles.
|
Table 6-3: Overview of the scenarios adopted for the climate
assessment.
|
|
Fixed-Year Scenario |
Name |
1992
|
2015
|
2050
|
Comments |
Fa1 |
NASA-1992*
|
NASA-2015*
|
FESGa
|
Technology option 1 |
Fa2 |
NASA-1992*
|
NASA-2015*
|
FESGa
|
Technology option 2 |
Fc1 |
NASA-1992*
|
NASA-2015*
|
FESGc
|
Technology option 1 |
Fe1 |
NASA-1992*
|
NASA-2015*
|
FESGe
|
Technology option 1 |
Eab |
NASA-1992*
|
EDF-a-base
|
EDF-a-base
|
|
Edh |
NASA-1992*
|
EDF-d-high
|
EDF-d-high
|
|
Fa1H
  |
NASA-1992*
 
|
NASA-2015*
 
|
FESGa+HSCT
 
|
Fa1 with part of subsonic traffic replaced by HSCT fleet growth from 2015
to 2040 |
|
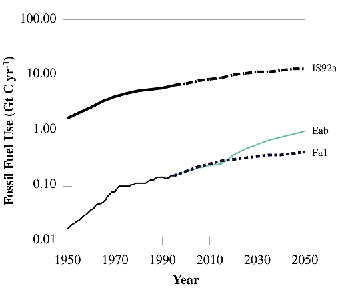
Figure 6-6: Fossil fuel use (Gt C yr-1) shown for
historical aviation use (1950-92, solid line) and for
projected aviation scenarios Fa1 and Eab. Total
historical fossil fuel use and the projection according
to scenario IS92a are also shown.
|
The total fuel in Gt C used by aviation from 1950 to 1992 is
shown in Figure 6-6. It also shows two projections
to 2050 (Fa1 and Eab; see Tables 6-1 and 6-2),
comparing them with projected total fossil carbon emissions for a similar economic
scenario (IS92a). Note the logarithmic scale in Figure
6-6. For scenario F1a, fuel use parallels that of IS92a, but for Eab it
grows faster than total fossil fuel use. In converting aviation fuel to CO2
emissions, we adopt a carbon fraction by weight of 86%. Aviation fuel use prior
to 1992 is based on International Energy Agency data (IEA, 1991; for table,
see Sausen and Schumann, 1999). To account for systematic underestimation of
fuel use (see Chapter 9), we have increased NASA-1992
and NASA-2015 emissions by 15% and 5%, respectively, to form the inventories
NASA-1992* and NASA-2015*. Figure 6-7 gives an expanded
linear scale of aviation fuel use from 1990 to 2050 for scenarios Fc1, Fa1,
Fa1H, Fe1, Eab, and Edh, in order of increasing fuel use by 2050.
Chapter 4 studies the impact of a fleet
of high-speed civil transport (HSCT, i.e., supersonic) aircraft using a range
of 3-D emission scenarios with atmospheric chemistry models. These calculations
form a parametric range that covers changes in fleet size, NOx emissions, cruise
altitude, sulfate aerosol formation, and future atmospheres. The present chapter
combines those results into a continuous scenario for the HSCT fleet, designated
Fa1H: On top of the Fa1 scenario it assumes that HSCT aircraft come into service
in 2015, grow at 40 planes per year to a final capacity of 1,000 aircraft by
2040, continue operation to 2050, and displace equivalent air traffic from the
subsonic fleet (~11% of Fa1 in 2050). This Mach 2.4 HSCT fleet cruises at 18-20
km altitude and deposits most of its emissions in the stratosphere. It has new
combustor technology that produces very low emissions of 5 g NO2
per kg fuel. Table 6-1 gives the breakdown of RF
from two specific HSCT studies in Chapter 4: 500 HSCTs
in a 2015 background atmosphere and 1,000 HSCTs in a 2050 background atmosphere
(e.g., chlorine loading, methane, nitrous oxide). The likely interval for the
RF here combines the uncertainty in calculating the ozone or water vapor perturbation
with that from calculating the radiative imbalance.
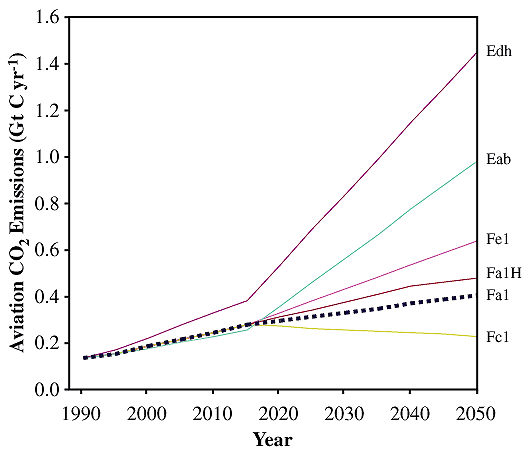
Figure 6-7: Aviation CO2 emissions (Gt C yr-1) from
1990 to 2050 for the range
of scenarios considered here
|
|