C.1 Observed Changes in Globally Well-Mixed Greenhouse Gas Concentrations
and Radiative Forcing
Over the millennium before the Industrial Era, the atmospheric concentrations
of greenhouse gases remained relatively constant. Since then, however, the concentrations
of many greenhouse gases have increased directly or indirectly because of human
activities.
Table 1 provides examples of several greenhouse
gases and summarises their 1750 and 1998 concentrations, their change during
the 1990s, and their atmospheric lifetimes. The contribution of a species to
radiative forcing of climate change depends on the molecular radiative properties
of the gas, the size of the increase in atmospheric concentration, and the residence
time of the species in the atmosphere, once emitted. The latter – the
atmospheric residence time of the greenhouse gas – is a highly policy relevant
characteristic. Namely, emissions of a greenhouse gas that has a long atmospheric
residence time is a quasi-irreversible commitment to sustained radiative forcing
over decades, centuries, or millennia, before natural processes can remove the
quantities emitted.
Table 1: Examples of greenhouse gases that are
affected by human activities. [Based upon Chapter 3
and Table 4.1] |
 |
|
CO2
(Carbon Dioxide) |
CH4
(Methane) |
N2O
(Nitrous Oxide) |
CFC-11
(Chlorofluoro-carbon-11) |
HFC-23
(Hydrofluoro-carbon-23) |
CF4
(Perfluoro-methane) |
 |
Pre-industrial concentration |
about 280 ppm |
about 700 ppb |
about 270 ppb |
zero |
zero |
40 ppt |
Concentration in 1998 |
365 ppm |
1745 ppb |
314 ppb |
268 ppt |
14 ppt |
80 ppt |
Rate of concentration change b |
1.5 ppm/yr a |
7.0 ppb/yr a |
0.8 ppb/yr |
-1.4 ppt/yr |
0.55 ppt/yr |
1 ppt/yr |
Atmospheric lifetime |
5 to 200 yr c |
12 yr d |
114 yr d |
45 yr |
260 yr |
>50,000 yr |
 |
Carbon dioxide (CO2)
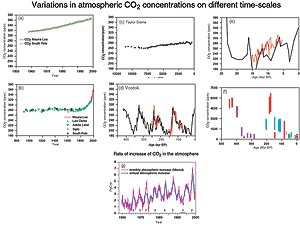
Figure 10: Variations in atmospheric CO2 concentration
on different time-scales. (a) Direct measurements of atmospheric CO2.
(b) CO2 concentration in Antarctic ice cores for the past millenium.
Recent atmospheric measurements (Mauna Loa) are shown for comparison. (c)
CO2 concentration in the Taylor Dome Antarctic ice core. (d)
CO2 concentration in the Vostok Antarctic ice core. (Different
colours represent results from different studies.) (e to f) Geochemically
inferred CO2 concentrations. (Coloured bars and lines represent
different published studies) (g) Annual atmospheric increases in CO2.
Monthly atmospheric increases have been filtered to remove the seasonal
cycle. Vertical arrows denote El Niño events. A horizontal line defines
the extended El Niño of 1991 to 1994. [Based on Figures
3.2 and 3.3] |
The atmospheric concentration of CO2 has increased from 280 ppm5
in 1750 to 367 ppm in 1999 (31%, Table 1). Today’s
CO2 concentration has not been exceeded during the past 420,000 years
and likely not during the past 20 million years. The rate of increase over the
past century is unprecedented, at least during the past 20,000 years (Figure
10). The CO2 isotopic composition and the observed decrease
in Oxygen (O2) demonstrates that the observed increase in CO2
is predominately due to the oxidation of organic carbon by fossil-fuel combustion
and deforestation. An expanding set of palaeo-atmospheric data from air trapped
in ice over hundreds of millennia provide a context for the increase in CO2
concentrations during the Industrial Era (Figure 10).
Compared to the relatively stable CO2 concentrations (280 ±
10 ppm) of the preceding several thousand years, the increase during the Industrial
Era is dramatic. The average rate of increase since 1980 is 0.4%/yr. The increase
is a consequence of CO2 emissions. Most of the emissions during the
past 20 years are due to fossil fuel burning, the rest (10 to 30%) is predominantly
due to land-use change, especially deforestation. As shown in Figure
9, CO2 is the dominant human-influenced greenhouse gas, with a
current radiative forcing of 1.46 Wm-2, being 60% of the total from
the changes in concentrations of all of the long-lived and globally mixed greenhouse
gases.
Direct atmospheric measurements of CO2 concentrations made over
the past 40 years show that year to year fluctuations in the rate of increase
of atmospheric CO2 are large. In the 1990s, the annual rates of CO2
increase in the atmosphere varied from 0.9 to 2.8 ppm/yr, equivalent to 1.9
to 6.0 PgC/yr. Such annual changes can be related statistically to short-term
climate variability, which alters the rate at which atmospheric CO2
is taken up and released by the oceans and land. The highest rates of increase
in atmospheric CO2 have typically been in strong El Niño years
(Box 4). These higher rates of increase can be plausibly
explained by reduced terrestrial uptake (or terrestrial outgassing) of CO2
during El Niño years, overwhelming the tendency of the ocean to take
up more CO2 than usual.
Partitioning of anthropogenic CO2 between atmospheric increases
and land and ocean uptake for the past two decades can now be calculated from
atmospheric observations. Table 2 presents a
global CO2 budget for the 1980s (which proves to be similar to the
one constructed with the help of ocean model results in the SAR) and for the
1990s. Measurements of the decrease in atmospheric oxygen (O2) as
well as the increase in CO2 were used in the construction of these
new budgets. Results from this approach are consistent with other analyses based
on the isotopic composition of atmospheric CO2 and with independent
estimates based on measurements of CO2 and 13CO2 in seawater.
The 1990s budget is based on newly available measurements and updates the budget
for 1989 to 1998 derived using SAR methodology for the IPCC Special Report on
Land Use, Land-Use Change and Forestry (2000). The terrestrial biosphere as
a whole has gained carbon during the 1980s and 1990s; i.e., the CO2
released by land-use change (mainly tropical deforestation) was more than compensated
by other terrestrial sinks, which are likely located in both the northern extra-tropics
and in the tropics. There remain large uncertainties associated with estimating
the CO2 release due to land-use change (and, therefore, with the
magnitude of the residual terrestrial sink).
Process-based modelling (terrestrial and ocean carbon models) has allowed
preliminary quantification of mechanisms in the global carbon cycle. Terrestrial
model results indicate that enhanced plant growth due to higher CO2
(CO2 fertilisation) and anthropogenic nitrogen deposition contribute
significantly to CO2 uptake, i.e., are potentially responsible for
the residual terrestrial sink described above, along with other proposed mechanisms,
such as changes in land-management practices. The modelled effects of climate
change during the 1980s on the terrestrial sink are small and of uncertain sign.
Table 2: Global CO2 budgets (in PgC/yr)
based on measurements of atmospheric CO2 and O2. Positive
values are fluxes to the atmosphere; negative values represent uptake from
the atmosphere. [Based upon Tables 3.1 and 3.3]
|
 |
|
SAR a,b
|
This Report a
|
 |
|
1980 to 1989
|
1980 to 1989
|
1990 to 1999
|
 |
Atmospheric increase |
3.3 ± 0.1
|
3.3 ± 0.1
|
3.2 ±0.1
|
Emissions (fossil fuel, cement) c |
5.5 ± 0.3
|
5.4 ± 0.3
|
6.3 ± 0.4
|
Ocean-atmosphere flux |
-2.0 ± 0.5
|
-1.9 ± 0.6
|
-1.7 ± 0.5
|
Land-atmosphere flux d |
-0.2 ± 0.6
|
-0.2 ± 0.7
|
-1.4 ± 0.7
|
 |
|