2.4 How Rapidly did Climate Change in the Distant Past?
2.4.1 Background
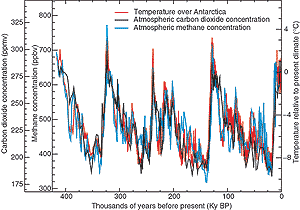
Figure 2.22: Variations of temperature, methane, and atmospheric carbon
dioxide concentrations derived from air trapped within ice cores from Antarctica
(adapted from Sowers and Bender, 1995; Blunier et al., 1997; Fischer et
al., 1999; Petit et al., 1999). |
Only during the 1980s was the possibility of rapid climatic changes occurring
at the time-scale of human life more or less fully recognised, largely due to
the Greenland ice core drilled at Dye 3 in Southern Greenland (Dansgaard et
al., 1982, 1989). A possible link between such events and the mode of operation
of the ocean was then subsequently suggested (Oeschger et al., 1984; Broecker
et al., 1985; see Broecker, 1997, for a recent review). The SAR reviewed the
evidence of such changes since the peak of the last inter-glacial period about
120 ky BP (thousands of years Before Present). It concluded that: (1) large
and rapid climatic changes occurred during the last Ice Age and during the transition
towards the present Holocene; (2) temperatures were far less variable during
this latter period; and (3) suggestions that rapid changes may have also occurred
during the last inter-glacial required confirmation.
These changes are now best documented from ice core, deep-sea sediment and
continental records. Complementary and generally discontinuous information comes
from coral and lake level data. The time-scale for the Pleistocene deep-sea
core record is based on the orbitally tuned oxygen isotope record from marine
sediments (Martinson et al., 1987), constrained by two radiometrically dated
horizons, the peak of the last inter-glacial (about 124 ky BP) and the Brunhes/Matuyama
reversal of the Earth’s magnetic field at about 780 ky BP. 14C-dating
is also used in the upper 50 ky BP; the result is a deep-sea core chronology
believed to be accurate to within a few per cent for the last million years.
14C-dating is also used for dating continental records as well as
the counting of annual layers in tree rings and varved lake records, whereas
ice-core chron-ologies are obtained by combining layer counting, glaciological
models and comparison with other dated records. The use of globally representative
records, such as changes in continental ice volume recorded in the isotopic
composition of deep-sea sediments, or changes in atmospheric composition recorded
in air bubbles trapped in ice cores, now allow such local records to be put
into a global perspective. Studies still largely focus on the more recent glacial-interglacial
cycle (the last 120 to 130 ky). Table 2.4 is a guide to
terminology.
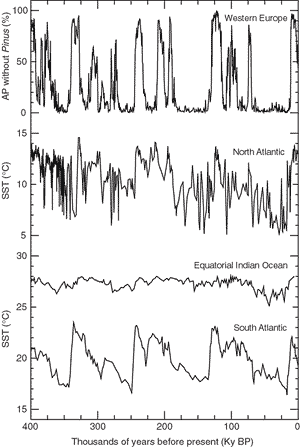
Figure 2.23: Time-series illustrating temperature variability over the
last about 400 ky (updated from Rostek et al., 1993; Schneider et al., 1996;
MacManus et al., 1999; Reille et al., 2000). The uppermost time-series describes
the percentage of tree pollen that excludes pollen from pine tree species.
The higher this percentage, the warmer was the climate. |
Before reviewing important recent information about rapid changes, we briefly
mention progress made on two aspects of the palaeoclimate record of relevance
for future climate. The first deals with the relationship between modern and
past terrestrial data and SSTs around the time of the Last Glacial Maximum (about
20 ky BP); this is important because of the use of glacial data to validate
climate models. New results obtained since the SAR both from marine and terrestrial
sources (reviewed in Chapter 8), agree on a tropical cooling
of about 3°C. The second concerns the greenhouse gas record (CO2
and CH4) which has now been considerably extended due to the recent
completion of drilling of the Vostok ice core in central East Antarctica. The
strong relationship between CO2 and CH4 and Antarctic
climate documented over the last climatic cycle has been remarkably confirmed
over four climatic cycles, spanning about 420 ky (Figure 2.22).
Present day levels of these two important greenhouse gases appear unprecedented
during this entire interval (Petit et al., 1999; and Figure
2.22). From a detailed study of the last three glacial terminations in the
Vostok ice core, Fischer et al. (1999) conclude that CO2 increases
started 600 ± 400 years after the Antarctic warming. However, considering
the large uncertainty in the ages of the CO2 and ice (1,000 years
or more if we consider the ice accumulation rate uncertainty), Petit et al.
(1999) felt it premature to ascertain the sign of the phase relationship between
CO2 and Antarctic temperature at the initiation of the terminations.
In any event, CO2 changes parallel Antarctic temperature changes
during deglaciations (Sowers and Bender, 1995; Blunier et al., 1997; Petit et
al., 1999). This is consistent with a significant contribution of these greenhouse
gases to the glacial-interglacial changes by amplifying the initial orbital
forcing (Petit et al., 1999).
We also now have a better knowledge of climate variability over the last few climatic
cycles as illustrated by selected palaeo-temperature records back to about 400
ky (Figure 2.23). The amplitude of the glacial-interglacial
temperature change was lower in tropical and equatorial regions (e.g., curve c)
than in mid- and high latitudes (other curves). During glacial periods, the climate
of the North Atlantic and adjacent regions (curves a and b) was more variable
than in the Southern Hemisphere (curve d). Also (not shown), full glacial periods
were characterised by very high fluxes of dust (seen in ice-core records and in
continental and marine records). A combination of increased dust source area,
stronger atmospheric transport and a weaker hydrological cycle (Yung et al., 1996;
Mahowald et al., 1999; Petit et al., 1999) probably generated these changes.
|