3.7.3.2 Concentration projections based on IS92a, for comparison with previous
studies
Illustrative model runs (Figure 3.11) based on the IS92a
scenario (Leggett et al., 1992) are shown first so as to allow comparison with
earlier model results presented in the SAR and the SRRF (Schimel et al., 1995).
In the SRRF comparison of eighteen global carbon cycle models (Enting et al.,
1994; Schimel et al., 1995) the CO2 fertilisation response of the
land was calibrated to match the central estimate of the global carbon budget
for the 1980s, assuming a land-use source of 1.6 PgC/yr in the 1980s and attributing
the residual terrestrial sink to CO2 fertilisation. This intercomparison
yielded CO2 concentrations in 2100 of 668 to 734 ppm; results presented
in Schimel et al. (1996) (from the Bern model) gave 688 ppm. After recalibrating
to match a presumed land-use source of 1.1 PgC/yr, implying a weaker CO2
response, the 2100 CO2 concentration was given as 712 ppm in the
SAR (Schimel et al., 1996). An IPCC Technical Paper (Wigley et al., 1997) evaluated
the sensitivity of IS92a results to this calibration procedure. Wigley et al.,
(1997) found that a range of assumed values from 0.4 to 1.8 PgC/yr for the land-use
source during the 1980s gave rise to a range of 2100 CO2 concentrations
from 667 to 766 ppm.
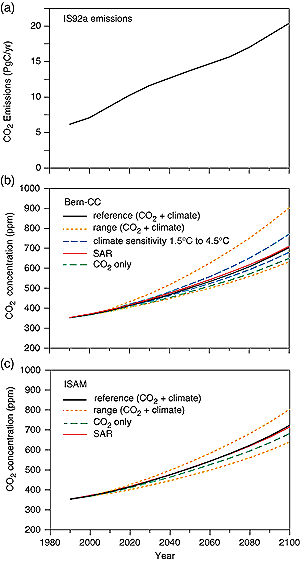
Figure 3.11: Projected CO2 concentrations resulting
from the IS92a emissions scenario. For a strict comparison with previous
work, IS92a-based projections were made with two fast carbon cycle models,
Bern-CC and ISAM (see Box 3.7), based on CO2
changes only, and on CO2 changes plus land and ocean climate
feedbacks. Panel (a) shows the CO2 emisisons prescribed by
IS92a; the panels (b) and (c) show projected CO2 concentrations
for the Bern-CC and ISAM models, respectively. Results obtained for the
SAR, using earlier versions of the same models, are also shown. The model
ranges for ISAM were obtained by tuning the model to approximate the range
of responses to CO2 and climate shown by the models in Figure
3.10, combined with a range of climate sensitivities from 1.5 to 4.5°C
rise for a doubling of CO2. This approach yields a lower bound
on uncertainties in the carbon cycle and climate. The model ranges for
Bern-CC were obtained by combining different bounding assumptions about
the behaviour of the CO2 fertilisation effect, the response
of heterotrophic respiration to temperature and the turnover time of the
ocean, thus approaching an upper bound on uncertainties in the carbon
cycle. The effect of varying climate sensitivity from 1.5 to 4.5°C
is shown separately for Bern-CC. Both models adopted a “reference
case” with mid-range behaviour of the carbon cycle and climate sensitivity
of 2.5°C.
|
In contrast with the SAR, the results presented here are based on approximating
the behaviour of spatially resolved process-based models in which CO2 and climate
responses are not constrained by prior assumptions about the global carbon budget.
The CO2-only response of both models’ reference cases (Figure
3.11) leads to a 2100 CO2 concentration of 682 ppm (ISAM) or 651 ppm (Bern-CC).
These values are slightly lower than projected in the SAR - 715 ppm (ISAM; SAR
version) and 712 ppm (Bern Model; SAR version) - because current process-based
terrestrial models typically yield a stronger CO2 response than was
assumed in the SAR. With climate feedbacks included, the 2100 CO2
concentration in the reference case becomes, by coincidence, effectively indistinguishable
from that given in the SAR: 723 ppm (ISAM) and 706 ppm (Bern-CC). The ranges
of 164 ppm or -12% / +11% (about the reference case) (ISAM) and 273 ppm or -10%
/ +28% (Bern-CC) in the 2100 CO2 concentration indicate that there
is significant uncertainty about the future CO2 concentrations due
to any one pathway of changes in emissions. Separate calculations with the Bern-CC
model (Figure 3.11) show that the effect of changing climate
sensitivity alone is less important than the effect of varying assumptions in
the carbon cycle model’s components. The effect of increasing climate sensitivity
to 4.5 °C (increasing the climate feedback) is much larger than the effect
of reducing climate sensitivity to 1.5 °C. The “low-CO2”
parametrization of Bern-CC yields CO2 concentrations closer to the
reference case than the “high-CO2” parametrization, in
which the terrestrial sink is forced to approach zero during the first few decades
of the century due to the capping of the CO2 fertilisation effect.
The reference simulations with ISAM yielded an implied average land-use source
during the 1980s of 0.9 PgC/yr. The range was 0.2 to 2.0 PgC/yr. Corresponding
values for Bern-CC were 0.6 PgC/yr and a range of 0.0 to 1.5 PgC/yr. These ranges
broadly overlap the range estimates of the 1980s land-use source given in Table
3.1. Present knowledge of the carbon budget is therefore not precise enough
to allow much narrowing of the uncertainty associated with future land and ocean
uptake as expressed in these projections. However, the lowest implied land-use
source values fall below the range given in Table 3.1.
Box 3.7: Fast, simplified models used in this assessment.
The Bern-CC model comprises:
- A box-diffusion type ocean carbon model, (HILDA version K(z); Siegenthaler
and Joos, 1992; Joos et al., 1996), already used in the SAR. In addition
to the SAR version, the effect of sea surface warming on carbonate chemistry
is included (Joos et al., 1999b).
- An impulse-response climate model (Hooss et al., 1999), which converts
radiative forcing into spatial patterns of changes in temperature, precipitation
and cloud cover on a global grid. The patterns of the climate anomalies
are derived from the first principal component of the climate response
shown by the full three-dimensional atmosphere-ocean GCM, ECHAM-3/LSG
(Voss and Mikolajewicz, 1999). Their magnitude is scaled according to
the prescribed climate sensitivity.
- The terrestrial carbon model LPJ, as described in Sitch et al. (2000)
and Cramer et al. (2001). LPJ is a process-based DGVM that falls in
the mid-range of CO2 and climate responses as shown in Cramer et al.
(2001). It is used here at 3.75° x 2.5° resolution, as in Cramer
et al. (2001).
- A radiative forcing module. The radiative forcing of CO2,
the concentration increase of non-CO2 greenhouse gases and
their radiative forcing, direct forcing due to sulphate, black carbon
and organic aerosols, and indirect forcing due to sulphate aerosols
are projected using a variant of SAR models (Harvey et al., 1997; Fuglestvedt
and Berntsen, 1999) updated with information summarised in Chapters
4, 5 and 6. The concentrations
of non-CO2 greenhouse gases, aerosol loadings, and radiative
forcings are consistent with those given in Appendix
II.
Sensitivities of projected CO2 concentrations to model assumptions
were assessed as follows. Rh was assumed either to be independent of global
warming (Giardina and Ryan, 2000; Jarvis and Linder 2000), or to increase
with temperature according to Lloyd and Taylor (1994). CO2
fertilisation was either capped after year 2000 by keeping CO2
at the year 2000 value in the photosynthesis module, or increased asymptotically
following Haxeltine and Prentice (1996). (Although apparently unrealistic,
capping the CO2 fertilisation in the model is designed to mimic
the possibility that other, transient factors such as land management
changes might be largely responsible for current terrestrial carbon uptake.)
Transport parameters of the ocean model (including gas exchange) were
scaled by a factor of 1.5 and 0.5. Average ocean uptake for the 1980s
is 2.0 PgC/yr in the reference case, 1.46 PgC/yr for the “slow ocean”
and 2.54 PgC/yr for the “fast ocean”, roughly in accord with
the range of observational estimates (Table 3.1,
Section 3.2.3.2). A “low-CO2”
parametrization was obtained by combining the fast ocean and no response
of Rh to temperature. A “high-CO2” parametrization
was obtained by combining the slow ocean and capping CO2 fertilisation.
Climate sensitivity was set at 2.5 °C for a doubling of CO2.
Effects of varying climate sensitivity from 1.5°C to 4.5°C are
also shown for one case.
The ISAM model was described by Jain et al. (1994) and used in the SAR
for CO2-only analyses, with a different set of model parameters
from those used here (Jain, 2000). The full configuration of ISAM comprises:
- A globally aggregated upwelling-diffusion ocean model including the
effects of temperature on CO2 solubility and carbonate chemistry
(Jain et al., 1995).
- An energy balance climate model of the type used in the IPCC 1990
assessment (Hoffert et al., 1980; Bretherton et al., 1990). In this
model, heat is transported as a tracer in the ocean and shares the same
transport parameters as DIC.
- A six-box globally aggregated terrestrial carbon model including empirical
parametrizations of CO2 fertilisation and temperature effects
on productivity and respiration (Harvey, 1989; Kheshgi et al., 1996).
- The radiative forcing of CO2 projected using a SAR model
(Harvey et al., 1997) modified with information summarised in Chapter
6. Radiative forcing from agents other than CO2 are identical
to that used in the Bern-CC model.
In addition to varying the climate sensitivity (1.5 to 4.5°C), parameters
of the terrestrial and ocean components (strength of CO2 fertilisation,
temperature response of NPP and heterotrophic respiration; ocean heat
and DIC transport) were adjusted to mimic the ranges of CO2
and climate responses as shown by existing process-based models (Figure
3.10). A reference case was defined with climate sensitivity 2.5°C,
ocean uptake corresponding to the mean of the ocean model results in Figure
3.10, and terrestrial uptake corresponding to the mean of the responses
of the mid-range models LPJ, IBIS and SDGVM (Figure
3.10). A “low CO2” parametrisation was chosen
with climate sensitivity 1.5°C, and maximal CO2 uptake
by oceans and land; and a “high-CO2” parametrization
with climate sensitivity 4.5°C, and minimal CO2 uptake
by oceans and land.
|
|