4. Natural and Human Systems
Natural and human systems are expected to be exposed to climatic variations
such as changes in the average, range, and variability of temperature and precipitation,
as well as the frequency and severity of weather events. Systems also would
be exposed to indirect effects from climate change such as sea-level rise, soil
moisture changes, changes in land and water condition, changes in the frequency
of fire and pest infestation, and changes in the distribution of infectious
disease vectors and hosts. The sensitivity of a system to these exposures depends
on system characteristics and includes the potential for adverse and beneficial
effects. The potential for a system to sustain adverse impacts is moderated
by adaptive capacity. The capacity to adapt human management of systems is determined
by access to resources, information and technology, the skill and knowledge
to use them, and the stability and effectiveness of cultural, economic, social,
and governance institutions that facilitate or constrain how human systems respond.
4.1. Water Resources
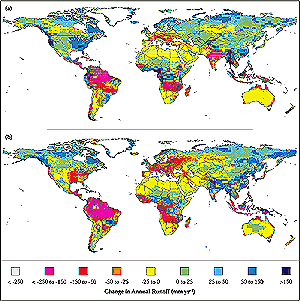
Figure TS-3: The pattern of changes in runoff largely follows the
pattern of simulated changes in precipitation, which varies between climate
models. The modeled increases in runoff shown in both maps [(a) HadCM2 ensemble
mean and (b) HadCM3; see Section 4.3.6.2 of Chapter
4 for discussion of models and scenarios used] for high latitudes and
southeast Asia, and decreases in central Asia, the area around the Mediterranean,
southern Africa, and Australia are broadly consistentin terms of direction
of changeacross most climate models. In other parts of the world,
changes in precipitation and runoff vary between climate change scenarios.
|
There are apparent trends in streamflow volumesincreases
and decreasesin many regions. However, confidence that these trends
are a result of climate change is low because of factors such as the variability
of hydrological behavior over time, the brevity of instrumental records, and
the response of river flows to stimuli other than climate change. In contrast,
there is high confidence that observations of widespread accelerated glacier
retreat and shifts in the timing of streamflow from spring toward winter in
many areas are associated with observed increases in temperature. High confidence
in these findings exists because these changes are driven by rising temperature
and are unaffected by factors that influence streamflow volumes. Glacier retreat
will continue, and many small glaciers may disappear (high confidence). The
rate of retreat will depend on the rate of temperature rise. [4.3.6.1,
4.3.11]
The effect of climate change on streamflow and groundwater recharge varies
regionally and among scenarios, largely following projected changes in precipitation.
In some parts of the world, the direction of change is consistent between scenarios,
although the magnitude is not. In other parts of the world, the direction of
change is uncertain. Possible streamflow changes under two climate change scenarios
are shown in Figure TS-3. Confidence in the projected
direction and magnitude of change in streamflow and groundwater recharge is
largely dependent on confidence in the projected changes in precipitation. The
mapped increase in streamflow in high latitudes and southeast Asia and the decrease
in streamflow in central Asia, the area around the Mediterranean, and southern
Africa are broadly consistent across climate models. Changes in other areas
vary between climate models. [4.3.5, 4.3.6.2]
Peak streamflow will move from spring to winter in many areas where snowfall
currently is an important component of the water balance (high confidence).
Higher temperatures mean that a greater proportion of winter precipitation falls
as rain rather than snow and therefore is not stored on the land surface until
it melts in spring. In particularly cold areas, an increase in temperature would
still mean that winter precipitation falls as snow, so there would be little
change in streamflow timing in these regions. The greatest changes therefore
are likely to be in "marginal" zonesincluding central and eastern Europe
and the southern Rocky Mountain chainwhere a small temperature rise reduces
snowfall substantially. [4.3.6.2]
Water quality generally would be degraded by higher water temperatures (high
confidence). The effect of temperature on water quality would be modified by
changes in flow volume, which may either exacerbate or lessen the effect of
temperature, depending on the direction of change in flow volume. Other
things being equal, increasing water temperature alters the rate of operation
of biogeochemical processes (some degrading, some cleaning) and, most important,
lowers the dissolved oxygen concentration of water. In rivers this effect may
be offset to an extent by increased streamflowwhich would dilute chemical
concentrations furtheror enhanced by lower streamflow, which would increase
concentrations. In lakes, changes in mixing may offset or exaggerate the effects
of increased temperature. [4.3.10]
Flood magnitude and frequency are likely to increase in most regions, and
low flows are likely to decrease in many regions. The general direction
of change in extreme flows and flow variability is broadly consistent among
climate change scenarios, although confidence in the potential magnitude of
change in any catchment is low. The general increase in flood magnitude and
frequency is a consequence of a projected general increase in the frequency
of heavy precipitation events, although the effect of a given change in precipitation
depends on catchment characteristics. Changes in low flows are a function of
changes in precipitation and evaporation. Evaporation generally is projected
to increase, which may lead to lower low flows even where precipitation increases
or shows little change. [4.3.8, 4.3.9]
Approximately 1.7 billion people, one-third of the world's population,
presently live in countries that are water-stressed (i.e., using more than 20%
of their renewable water supplya commonly used indicator of water stress).
This number is projected to increase to about 5 billion by 2025, depending on
the rate of population growth. Projected climate change could further decrease
streamflow and groundwater recharge in many of these water-stressed countriesfor
example, in central Asia, southern Africa, and countries around the Mediterranean
Seabut may increase it in some others.
Demand for water generally is increasing, as a result of population growth
and economic development, but is falling in some countries. Climate change
may decrease water availability in some water-stressed regions and increase
it in others. Climate change is unlikely to have a large effect on municipal
and industrial demands but may substantially affect irrigation withdrawals.
In the municipal and industrial sectors, it is likely that nonclimatic drivers
will continue to have very substantial effects on demand for water. Irrigation
withdrawals, however, are more climatically determined, but whether they increase
or decrease in a given area depends on the change in precipitation: Higher temperatures,
hence crop evaporative demand, would mean that the general tendency would be
toward an increase in irrigation demands. [4.4.2, 4.4.3,
4.5.2]
The impact of climate change on water resources depends not only on changes
in the volume, timing, and quality of streamflow and recharge but also on system
characteristics, changing pressures on the system, how management of the system
evolves, and what adaptations to climate change are implemented. Nonclimatic
changes may have a greater impact on water resources than climate change.
Water resources systems are evolving continually to meet changing management
challenges. Many of the increased pressures will increase vulnerability to climate
change, but many management changes will reduce vulnerability. Unmanaged systems
are likely to be most vulnerable to climate change. By definition, these systems
have no management structures in place to buffer the effects of hydrological
variability. [4.5.2]
Climate change challenges existing water resources management practices
by adding uncertainty. Integrated water resources management will enhance the
potential for adaptation to change. The historic basis for designing and
operating infrastructure no longer holds with climate change because it cannot
be assumed that the future hydrological regime will be the same as that of the
past. The key challenge, therefore, is incorporating uncertainty into water
resources planning and management. Integrated water resources management is
an increasingly used means of reconciling different and changing water uses
and demands, and it appears to offer greater flexibility than conventional water
resources management. Improved ability to forecast streamflow weeks or months
ahead also would significantly enhance water management and its ability to cope
with a changing hydrological variability. [4.6]
Adaptive capacity (specifically, the ability to implement integrated water
resources management), however, is very unevenly distributed across the world.
In practice, it may be very difficult to change water management practices in
a country where, for example, management institutions and market-like processes
are not well developed. The challenge, therefore, is to develop ways to introduce
integrated water management practices into specific institutional settingswhich
is necessary even in the absence of climate change to improve the effectiveness
of water management. [4.6.4]
|