Options to Limit or Reduce Greenhouse Gas Emissions
and Enhance Sinks
7. Significant technical progress relevant to greenhouse
gas emissions reduction has been made since the SAR in 1995 and has been faster
than anticipated. Advances are taking place in a wide range of technologies
at different stages of development, e.g., the market introduction of wind turbines,
the rapid elimination of industrial by-product gases such as N2O
from adipic acid production and perfluorocarbons from aluminium production,
efficient hybrid engine cars, the advancement of fuel cell technology, and the
demonstration of underground carbon dioxide storage. Technological options for
emissions reduction include improved efficiency of end use devices and energy
conversion technologies, shift to low-carbon and renewable biomass fuels, zero-emissions
technologies, improved energy management, reduction of industrial by-product
and process gas emissions, and carbon removal and storage (Section 3.1,
4.7).
Table SPM.1 summarizes the results from many
sectoral studies, largely at the project, national and regional level with some
at the global levels, providing estimates of potential greenhouse gas emission
reductions in the 2010 to 2020 timeframe. Some key findings are:
- Hundreds of technologies and practices for end-use energy efficiency in
buildings, transport and manufacturing industries account for more than half
of this potential (Sections 3.3, 3.4,
3.5).
- At least up to 2020, energy supply and conversion will remain dominated
by relatively cheap and abundant fossil fuels. Natural gas, where transmission
is economically feasible, will play an important role in emission reduction
together with conversion efficiency improvement, and greater use of combined
cycle and/or co-generation plants (Section 3.8.4).
- Low-carbon energy supply systems can make an important contribution through
biomass from forestry and agricultural by-products, municipal and industrial
waste to energy, dedicated biomass plantations, where suitable land and water
are available, landfill methane, wind energy and hydropower, and through the
use and lifetime extension of nuclear power plants. After 2010, emissions
from fossil and/or biomass-fueled power plants could be reduced substantially
through pre- or post-combustion carbon removal and storage. Environmental,
safety, reliability and proliferation concerns may constrain the use of some
of these technologies (Section 3.8.4).
- In agriculture, methane and nitrous oxide emissions can be reduced, such
as those from livestock enteric fermentation, rice paddies, nitrogen fertilizer
use and animal wastes (Section 3.6).
- Depending on application, emissions of fluorinated gases can be minimized
through process changes, improved recovery, recycling and containment, or
avoided through the use of alternative compounds and technologies (Section
3.5 and Chapter 3 Appendix).
The potential emissions reductions found in Table
SPM.1 for sectors were aggregated to provide estimates of global potential
emissions reductions taking account of potential overlaps between and within
sectors and technologies to the extent possible given the information available
in the underlying studies. Half of these potential emissions reductions may
be achieved by 2020 with direct benefits (energy saved) exceeding direct costs
(net capital, operating, and maintenance costs), and the other half at a net
direct cost of up to US$100/tCeq (at 1998 prices). These cost estimates are
derived using discount rates in the range of 5% to 12%, consistent with public
sector discount rates. Private internal rates of return vary greatly, and are
often significantly higher, affecting the rate of adoption of these technologies
by private entities.
Depending on the emissions scenario this could allow global emissions to be
reduced below 2000 levels in 20102020 at these net direct costs. Realizing
these reductions involve additional implementation costs, which in some cases
may be substantial, the possible need for supporting policies (such as those
described in Paragraph 18), increased research and
development, effective technology transfer and overcoming other barriers (Paragraph
17). These issues, together with costs and benefits not included in this
evaluation are discussed in Paragraphs 11, 12
and 13.
The various global, regional, national, sector and project studies assessed
in this report have different scopes and assumptions. Studies do not exist for
every sector and region. The range of emissions reductions reported in Table
SPM.1 reflects the uncertainties (see Box SPM.2)
of the underlying studies on which they are based (Sections
3.3-3.8)
Box SPM.1. The Emissions Scenarios of the IPCC Special Report on
Emissions Scenarios (SRES
A1. The A1 storyline and scenario family describes a future world
of very rapid economic growth, global population that peaks in mid-century
and declines thereafter, and the rapid introduction of new and more
efficient technologies. Major underlying themes are convergence among
regions, capacity building and increased cultural and social interactions,
with a substantial reduction in regional differences in per capita income.
The A1 scenario family develops into three groups that describe alternative
directions of technological change in the energy system. The three A1
groups are distinguished by their technological emphasis: fossil intensive
(A1FI), non-fossil energy sources (A1T), or a balance across all sources
(A1B) (where balanced is defined as not relying too heavily on one particular
energy source, on the assumption that similar improvement rates apply
to all energy supply and end use technologies).
A2. The A2 storyline and scenario family describes a very heterogeneous
world. The underlying theme is self-reliance and preservation of local
identities. Fertility patterns across regions converge very slowly,
which results in continuously increasing population. Economic development
is primarily regionally oriented and per capita economic growth and
technological change more fragmented and slower than other storylines.
B1. The B1 storyline and scenario family describes a convergent world
with the same global population, that peaks in mid-century and declines
thereafter, as in the A1 storyline, but with rapid change in economic
structures toward a service and information economy, with reductions
in material intensity and the introduction of clean and resource-efficient
technologies. The emphasis is on global solutions to economic, social
and environmental sustainability, including improved equity, but without
additional climate initiatives.
B2. The B2 storyline and scenario family describes a world in which
the emphasis is on local solutions to economic, social and environmental
sustainability. It is a world with continuously increasing global population,
at a rate lower than A2, intermediate levels of economic development,
and less rapid and more diverse technological change than in the B1
and A1 storylines. While the scenario is also oriented towards environmental
protection and social equity, it focuses on local and regional levels.
An illustrative scenario was chosen for each of the six scenario groups
A1B, A1FI, A1T, A2, B1 and B2. All should be considered equally sound.
The SRES scenarios do not include additional climate initiatives, which
means that no scenarios are included that explicitly assume implementation
of the United Nations Framework Convention on Climate Change or the
emissions targets of the Kyoto Protocol.
|
8. Forests, agricultural lands, and other terrestrial ecosystems
offer significant carbon mitigation potential. Although not necessarily permanent,
conservation and sequestration of carbon may allow time for other options to
be further developed and implemented. Biological mitigation can occur by
three strategies: (a) conservation of existing carbon pools, (b) sequestration
by increasing the size of carbon pools, and (c) substitution of sustainably
produced biological products, e.g. wood for energy intensive construction products
and biomass for fossil fuels (Sections 3.6, 4.3).
Conservation of threatened carbon pools may help to avoid emissions, if leakage
can be prevented, and can only become sustainable if the socio-economic drivers
for deforestation and other losses of carbon pools can be addressed. Sequestration
reflects the biological dynamics of growth, often starting slowly, passing through
a maximum, and then declining over decades to centuries.
Conservation and sequestration result in higher carbon stocks, but can lead
to higher future carbon emissions if these ecosystems are severely disturbed
by either natural or direct/indirect human-induced disturbances. Even though
natural disturbances are normally followed by re-sequestration, activities to
manage such disturbances can play an important role in limiting carbon emissions.
Substitution benefits can, in principle, continue indefinitely. Appropriate
management of land for crop, timber and sustainable bio-energy production, may
increase benefits for climate change mitigation. Taking into account competition
for land use and the SAR and SRLULUCF assessments, the estimated global potential
of biological mitigation options is in the order of 100GtC (cumulative), although
there are substantial uncertainties associated with this estimate, by 2050,
equivalent to about 10% to 20% of potential fossil fuel emissions during that
period. Realization of this potential depends upon land and water availability
as well as the rates of adoption of different land management practices. The
largest biological potential for atmospheric carbon mitigation is in subtropical
and tropical regions. Cost estimates reported to date of biological mitigation
vary significantly from US$0.1/tC to about US$20/tC in several tropical countries
and from US$20/tC to US$100/tC in non-tropical countries. Methods of financial
analysis and carbon accounting have not been comparable. Moreover, the cost
calculations do not cover, in many instances, inter alia, costs for infrastructure,
appropriate discounting, monitoring, data collection and implementation costs,
opportunity costs of land and maintenance, or other recurring costs, which are
often excluded or overlooked. The lower end of the ranges are biased downwards,
but understanding and treatment of costs is improving over time. These biological
mitigation options may have social, economic and environmental benefits beyond
reductions in atmospheric CO2, if implemented appropriately. (e.g.,
biodiversity, watershed protection, enhancement of sustainable land management
and rural employment). However, if implemented inappropriately, they may pose
risks of negative impacts (e.g., loss of biodiversity, community disruption
and ground-water pollution). Biological mitigation options may reduce or increase
non-CO2 greenhouse gas emissions (Sections 4.3,
4.4).
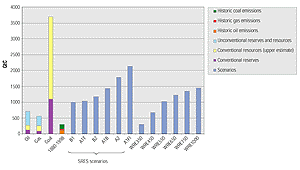 |
Figure SPM.2: Carbon in oil, gas and coal reserves
and resources compared with historic fossil fuel carbon emissions 18601998,
and with cumulative carbon emissions from a range of SRES scenarios and
TAR stabilization scenarios up until 2100. Data for reserves and resources
are shown in the left hand columns ( Section 3.8.2).
Unconventional oil and gas includes tar sands, shale oil, other heavy
oil, coal bed methane, deep geopressured gas, gas in acquifers, etc. Gas
hydrates (clathrates) that amount to an estimated 12,000GtC are not shown.
The scenario columns show both SRES reference scenarios as well as scenarios
which lead to stabilization of CO 2 concentrations at a range
of levels. Note that if by 2100 cumulative emissions associated with SRES
scenarios are equal to or smaller than those for stabilization scenarios,
this does not imply that these scenarios equally lead to stabilization.
|
|