10.4.1.2 Climate variability and extreme events
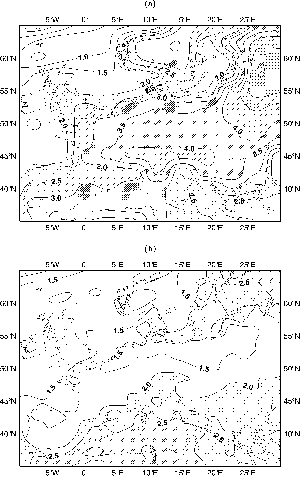
Figure 10.9: Winter surface air temperature change (°C) over
Europe at the time of CO2 doubling in (a) a transient climate
change experiment with the AOGCM HadCM2 and (b) the stretched grid AGCM
ARPEGE driven by SSTs and sea-ice from the HadCM2 integration. From Dèquè
et al. (1998). |
Enhanced resolution improves many aspects of the AGCMs’
intra-seasonal variability of circulation at low and intermediate frequencies
(Stendel and Roeckner, 1998). However, in some cases values underestimated at
standard resolution are overestimated at enhanced resolution (Dèquè
and Piedelievre, 1995; Stratton, 1999a,b). Martin (1999) found little sensitivity
to resolution in either the interannual or intra-seasonal variability of circulation
and precipitation of the South Asian monsoon in HadAM3a. Extreme events have
not been studied, with the exception of tropical cyclones. This subject cuts
across various sections and chapters and thus is dealt with in Box
10.2.
10.4.2 Simulations of Climate Change
10.4.2.1 Mean climate
Climate change simulations using ECHAM3 at T42 and T106 resolutions predicted
substantially different responses for southern Europe (Cubash et al., 1996).
For example, surface temperature response of less than +2ºC in summer at
T42 increased by over 4ºC for much of the region at T106 and winter precipitation
increased more at T106 than at T42. An important factor in generating the different
responses was the substantial difference in the control simulations. Wild et
al. (1997) showed a large positive summer surface temperature bias in the T106
control derived from a positive feedback between excessive surface insolation
and summer dryness. This mechanism provided a large increase in the insolation,
and thus the surface temperature, in the anomaly experiment. As this process
was handled poorly in the control simulation, little confidence can be placed
in the warming amplification simulated at T106.
A variable grid AGCM climate change experiment using the ARPEGE model and sea
surface forcing from HadCM2 predicted moderate warmings over Europe, 1.5ºC
(northern) to 2.5ºC (southern) in winter and 1ºC to 3.5ºC in
summer (Dèquè et al., 1998). In contrast, HadCM2 predicted greater
warming and a larger north-south gradient in winter (Figure
10.9). These differences result mainly from the ARPEGE large-scale flow
being too zonal and too strong over mainland Europe, which enhances the moderating
influence of the SSTs. The precipitation responses are more similar, especially
in summer, when both models predict a decrease over most of Europe, maximum
–30% in the south. Differences in the control simulations suggest that
little confidence should be placed in this result.
In a similar experiment, HadAM3a at 1.25°30.83° resolution used observed
sea surface forcing and anomalies from a HadCM3 GHG simulation and produced
a response at the largest scales in the annual mean similar to the AOGCM (Johns,
1999). However, regionally or seasonally, many differences were evident in the
two models, notably in land sea contrasts, monsoon precipitation and some circulation
features. Over Europe, large-scale responses in surface temperature and precipitation
were similar except for a larger winter surface warming in northern Europe in
HadCM3. This was due to a greater melting back of Arctic sea ice which was too
extensive in the HadCM3 control (Jones, 1999). In a 30-year ECHAM4 T106 experiment
driven by ECHAM4/OPYC simulations for 1970 to 1999 and 2060 to 2089, the simulations
of future climate were more similar to each other than those for the present
day (May, 1999). This implies that the differences in the control simulations
would determine a proportion of the difference in the responses. In these cases
better control simulations at high resolution increase the confidence in their
responses.
10.4.2.2 Climate variability and extreme events
Due to the limited number and length of simulations and a lack of comprehensive
analyses, this subject has been almost completely ignored. The only response
in variability or extremes that has received any attention is that of tropical
cyclones (Box 10.2).
Box 10.2: Tropical cyclones in current and future
climates
Simulating a climatology of tropical cyclones
Tropical cyclones can have devastating human and economic impacts (e.g.,
Pielke and Landsea, 1998) and therefore accurate estimates of future changes
in their frequency, intensity and location would be of great value. However,
because of their relatively small extent (in global modelling terms) and
intense nature, detailed simulation of tropical cyclones for this purpose
is difficult. Atmospheric GCMs can simulate tropical cyclone-like disturbances
which increase in realism at higher resolution though the intense central
core is not resolved (e.g., Bengtsson et al., 1995; McDonald, 1999). Further
increases of resolution, by the use of RCMs, provide greater realism (e.g.,
Walsh and Watterson, 1997) with a very high resolution regional hurricane
prediction model giving a reasonable simulation of the magnitude and location
of maximum surface wind intensities for the north-west Pacific basin (Knutson
et al., 1998). GCMs generally provide realistic simulation of the location
and frequency of tropical cyclones (e.g., Tsutsui and Kasahara, 1996;
Yoshimura et al., 1999). See also Chapter 8 for
more details on tropical cyclones in GCMs.
Tropical cyclones in a warmer climate
Much effort has gone into obtaining and analysing good statistics on tropical
cyclones in the recent past. The main conclusion is that there is large
decadal variability in the frequency and no significant trend during the
last century. One study looking at the century time-scale has shown an
increase in the frequency of North Atlantic cyclones from 1851 to 1890
and 1951 to 1990 (Fernandez-Partagas and Diaz, 1996). See Chapter
2 for more details on observed tropical cyclones.
Most assessments of changes in tropical cyclone behaviour in a future
climate have been derived from GCM or RCM studies of the climate response
to anthropogenically-derived atmospheric forcings (e.g., Bengtsson et
al., 1996, 1997; Walsh and Katzfey, 2000). Recently, more focused approaches
have been used: nesting a hurricane prediction model in a GCM climate
change simulation (Knutson et al., 1998); inserting idealised tropical
cyclones into an RCM climate change simulation (Walsh and Ryan, 2000).
In an early use of a high-resolution AGCM, a T106 ECHAM3 experiment simulated
a decrease in tropical cyclones in the Northern Hemisphere and a reduction
of 50% in the Southern Hemisphere (Bengtsson et al., 1996, 1997). However,
the different hemispheric responses raised questions about the model’s
ability to properly represent tropical cyclones and methodological concerns
about the experimental design were raised (Landsea, 1997). In a similar
experiment, the JMA model also simulated fewer tropical cyclone-like vortices
in both hemispheres (Yoshimura et al., 1999). Other GCM studies have shown
consistent basin-dependent changes in tropical cyclone formation under
2xCO2 conditions (Royer et al., 1998; Tsutsui et al., 1999).
Frequencies increased in the north-west Pacific, decreased in the North
Atlantic, and changed little in the south-west Pacific. A high resolution
HadAM3a simulation reproduced the latter changes, giving changes in timing
in the north-west Pacific and increases in frequency in the north-east
Pacific and the north Indian basin (McDonald, 1999). Some GCM studies
show increases in tropical storm intensity in a warmer climate (Krishnamurti
et al., 1998) though these results can probably not be extrapolated to
tropical cyclones as the horizontal resolution of these models is insufficient
to resolve the cyclone eye. The likely mean response of tropical Pacific
sea surface warming having an El Niño-like structure suggests that
the pattern of tropical cyclone frequency may become more like that observed
in El Niño years (see Chapter 9).
An indication of the likely changes in maximum intensity of cyclones
will be better provided by models able to simulate realistic tropical
cyclone intensities. A sample of GCM-generated tropical cyclone cases
nested in a hurricane prediction model gave increases in maximum intensity
(of wind speed) of 5 to 11% in strong cyclones over the north-west Pacific
for a 2.2°C SST warming (Knutson and Tuleya, 1999). The RCM study
of idealised tropical cyclones (in the South Pacific) showed a small,
but not statistically significant, increase in maximum intensity (Walsh
and Ryan, 2000). These results are supported by the theory of the maximum
potential intensity (MPI) of hurricanes (Emanuel, 1987). A calculation
using the MPI framework of Holland (1997) suggested increases of 10 to
20% for a 2xCO2 climate (Henderson-Sellers et al., 1998). This study also
acknowledges physical omissions that would reduce this estimate though
Emanuel (2000) suggests there is a linear relationship betweeen MPI and
the wind speed of real events. Published modelling studies to date neglect
the possible feedback of sea surface cooling induced by the cyclone. However,
a recently submitted study using a hurricane model with ocean coupling
indicates that the increased maximum intensity by CO2 warming
would still occur even when the sea surface cooling feedback is included
(Knutson et al., 2000).
The extreme precipitation associated with tropical cyclones can also
be very damaging. The very high resolution studies discussed above suggest
that increases in the intensity of tropical cyclones will be accompanied
by increases in mean and maximum precipitation rates. In the cases studied,
precipitation in the vicinity of the storm centre increased by 20% whereas
peak rates increased by 30%. Part of these increases may be due to the
increased moisture-holding capacity of a warmer atmosphere but nevertheless
point to substantially increasing destructive capacity of tropical cyclones
in a warmer climate.
In conclusion, there is some evidence that regional frequencies of
tropical cyclones may change but none that their locations will change.
There is also evidence that the peak intensity may increase by 5% to 10%
and precipitation rates may increase by 20% to 30%. There is a need for
much more work in this area to provide more robust results.
|
10.4.3 Summary and Recommendations
Since the SAR, several variable and high-resolution GCMs have been used to
provide high-resolution simulations of climate change. Clearly the technique
is still in its infancy with only a few modelling studies carried out and for
only a limited number of regions. Also, there is little in-depth analysis of
the performance of the models and only preliminary conclusions can be drawn.
Many aspects of the models’ dynamics and large-scale flow are improved
at higher resolution, though this is not uniformly so geographically or across
models. Some models also demonstrate improvements in their surface climatologies
at higher resolution. However, substantial underlying errors are often still
present in high-resolution versions of current AGCMs. In addition, the direct
use of high-resolution versions of current AGCMs, without some allowance of
the dependence of models physical parametrizations on resolution, leads to some
deterioration in the performance of the models.
Regional responses currently appear more sensitive to the AGCM than the SST
forcing used. This result is partially due to some of the model responses being
dependent on their control simulations and systematic errors within them. These
factors and the small number of studies carried out imply that little confidence
can be attached to any of the regional projections provided by high and variable
resolution AGCM simulations. The improvements seen with this technique are encouraging,
but more effort should be put in analysing, and possibly improving the performance
of current models at high resolution. This is particularly important in view
of the fact that future AOGCMs will likely use models approaching the resolution
considered here in the next 5 to 10 years.
|